Key Concepts
The capability that a bacterium acquires to counteract an inhibitory chemical molecule or compound that was formerly effective in killing it or preventing its growth. Antibiotic resistance in bacteria (Fig. 1) is a hot topic in the media and in scientific and health care settings worldwide. The U.S. Centers for Disease Control and Prevention (CDC) describes antibiotic-resistant bacteria as “nightmare bacteria” that pose a catastrophic threat to people in every country in the world. It is estimated that 2 million people in the United States become infected by resistant bacteria each year. The CDC indicates that at least 23,000 people die per year as a direct result of antibiotic-resistant infections, with more individuals dying because of complications that occur as a result of these infections. The CDC classifies three types of infections as urgent threats to public health: (1) carbapenem-resistant Enterobacteriaceae (CRE); (2) drug-resistant gonorrhea; and (3) Clostridioides difficile (formerly, Clostridium difficile), which is linked to serious diarrheal illnesses associated with antibiotic use. The current status of antibiotic resistance worldwide needs to be investigated meticulously, and ways must be found to halt its progression. See also: Antibiotic; Antimicrobial agents; Bacteria; Bacteriology; Drug resistance; Infection; Medical bacteriology; Microbiology; Public health
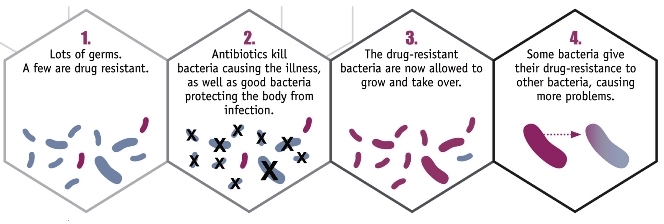
Identification of antibiotics
Antibiotics were first identified by Alexander Fleming in 1928, in what amounted to an accidental discovery. Fleming was working with the bacteria pathogen Staphylococcus aureus; he returned from a long holiday to discover that the growth on some of his plates had been inhibited by a mold that was also growing on the plates. This mold was Penicillium, and the substance that it produced was named “penicillin” by Fleming. See also: Penicillin; Staphylococcus
Although this was the first time that a scientist was able to visualize the effect of an antibiotic on bacteria, many human populations had employed mold to treat infections. For example, peasants in Eastern Europe kept a moldy loaf of bread wrapped in a moist towel, typically in the rafters of their houses. When an injury to a person occurred, a paste was made from the moldy bread and applied to the wound to prevent infection from developing.
First development of resistance
Although Fleming discovered penicillin in 1928, it took more than 13 years to develop a method of mass-producing the drug; by 1944, it was in widespread use to treat wounded soldiers during World War II. Within four years of its initial use, however, strains of S. aureus started to appear that were resistant to the antibiotic. Today, nearly every strain of S. aureus seen in the clinical setting makes penicillinase, an enzyme that breaks down the antibiotic, and therefore penicillin cannot be used to treat infections caused by this microbial species. See also: Enzyme
As resistance to penicillin grew, scientists began to develop alternative antibiotics. Methicillin (also spelled meticillin) was developed in Britain in 1959 to treat infections caused by susceptible Gram-positive bacteria (that is, bacteria that show a positive reaction, and turn purple, when a Gram staining test is carried out). Methicillin was resistant to penicillinases, making it effective against species that could produce the enzyme. However, by 1961, methicillin-resistant strains of S. aureus (MRSA) had appeared in British hospitals; by 1968, it had appeared in the United States. The percentage of hospital-acquired (HA) infections (nosocomial infections) caused by MRSA strains rather than susceptible strains rose gradually in the United States, reaching 50% by 1997. Community-acquired (CA) strains of MRSA started to appear in 1981, with a number of children without underlying disease becoming infected. In 1999, the first deaths resulting from CA-MRSA in otherwise healthy children were reported. See also: Human susceptibility to Staphylococcus aureus; Methicillin-resistant Staphylococcus aureus (MRSA); Nosocomial disease; Staining
Modern issues
Two of the major players in the rise of antibiotic resistance are Clostridioides difficile and Gram-negative bacteria.
Clostridioides difficile
Clostridioides difficile (formerly, Clostridium difficile) is a Gram-positive, endospore-forming, anaerobic, rod-shaped bacterium. It is typically found in soil and in the intestinal tract of many mammals, including humans. In addition, it is found in low numbers in the intestinal tract of carriers; when antibiotics are taken by a carrier, the other, more susceptible species in the gut die off, leaving behind a vacuum that C. difficile can then fill and causing what is known as a “superinfection” (Fig. 2). Resistance is variable among C. difficile isolates; most infections can be treated using vancomycin or metronidazole, although resistance has been seen in a number of countries. Individuals who are already hospitalized comprise the most at-risk group for this disease, and this type of resistance occurs at a rate of 4 to 8 patients per 1000. According to the CDC, 250,000 new hospitalizations occur each year as a result of this disease, with at least 14,000 deaths. See also: Antibiotic resistance in soil bacteria; Clostridium; Human microbiota; Microbiome; Soil microbiology
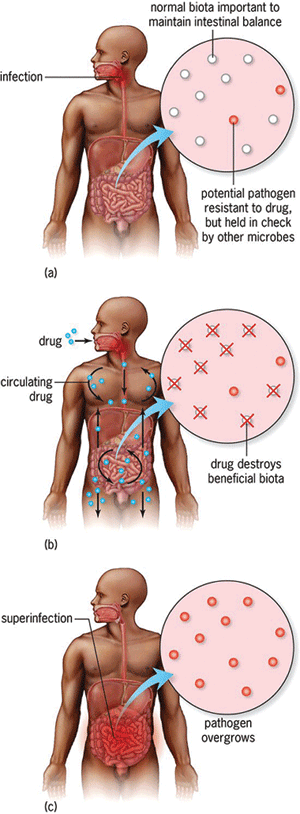
Gram-negative superbugs
Strains of the carbapenem-resistant Enterobacteriaceae (CRE), known in the media as the “Gram-negative superbugs,” were very rare in the United States before the 1990s. Carbapenem is a member of the beta-lactam group of antibiotics, which also includes penicillin; these antibiotics inhibit the synthesis of peptidoglycan, the substance that makes up the bacterial cell wall. Compared to penicillin, though, carbapenem has a broader spectrum of activity and therefore has better activity against Gram-negative species. However, strains of CRE, which include strains of Escherichia coli, Klebsiella pneumoniae, Klebsiella oxytoca, Enterobacter cloacae, and Enterobacter aerogenes, have evolved mechanisms by which they can inactivate carbapenem, making them resistant to the drug. These species are typically found as commensals in the intestinal tract of healthy people. Given the right opportunity, though, such as might occur in a hospital setting, they can result in infection and disease.
Hospitals are the usual transmission site for infections caused by CRE. Most individuals who develop CRE-based infections are in long-term care or have other, underlying compromised conditions, such as being diabetic or requiring mechanical ventilation. CRE strains are typically passed via some type of fomite or inanimate object; these can include surfaces in patient rooms and medical equipment that has not been properly disinfected after patient use.
Treatment of CRE infections involves using alternative antibiotics, including fosfomycin, tigecycline, nitrofurantoin, pivmecillinam, and colistin. Colistin, an antibiotic classified as a polymixin that has been in use since 1959, is frequently the last drug available to treat these superbug infections. Now, however, the situation is changing; in late 2015, colistin-resistant CRE strains were found in food products in China. Specifically, these bacteria were discovered in raw pork and chicken that were packaged for sale. Since then, a colistin-resistant CRE strain has been found in a sample taken from a pig in the United States, and another CRE strain was found in the urine of a woman in Pennsylvania, alarming the CDC and other scientists.
Resistance mechanisms
Resistance to bacteria can arise as a result of a variety of mechanisms that occur in bacterial cells. Typically, bacteria evolve under the pressure of antibiotics in their environment. The primary mechanisms of evolution resulting in resistance occur through spontaneous mutations or through genetic exchanges among bacterial cells. In addition, some bacteria are intrinsically or naturally resistant to certain antibiotics. For example, Mycoplasma pneumoniae is resistant to penicillin and other antibiotics that target the cell wall and its synthesis. This is the case because this species does not actually have a cell wall, and therefore the antibiotics are completely ineffective. See also: Bacterial genetics; Mutation; Mycoplasmas
Mutations that occur in a particular bacterial cell are subsequently inherited by daughter cells. These mutations can be acquired randomly; however, advantageous mutations under conditions of environmental stress, including increased antibiotic concentrations, will encourage their continuance in the population (Fig. 3). Antibiotics are administered frequently in a hospital setting, often using a syringe for administration. When injected, a small amount may be aerosolized to ensure that bubbles are not present; this leads to higher concentrations of the antibiotic in the environment, which can influence resistance development.
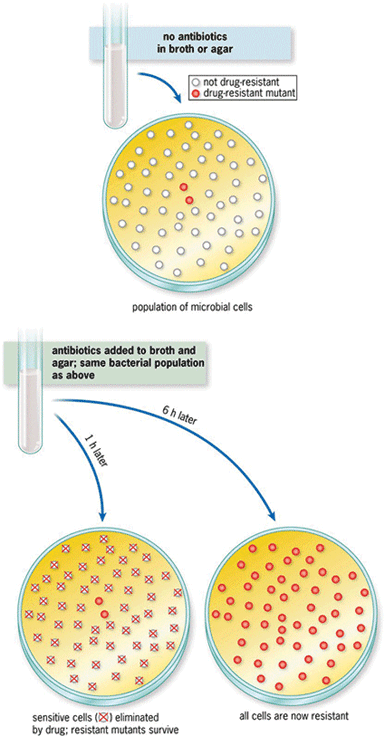
In addition to mutation, resistance may be passed between bacterial cells via horizontal gene transfer. This involves either conjugation [the passage of deoxyribonucleic acid (DNA) between cells through a long, narrow tube known as a sex pilus] or transformation (uptake of DNA from a cell that has lysed or disintegrated). There are five main resistance mechanisms that can occur in bacteria (Fig. 4): (1) inactivation of the antibiotic, such as through production of a beta-lactamase (which breaks down beta-lactam antibiotics); (2) alteration of the drug target site, including the penicillin-binding site, thereby making any drugs ineffective; (3) alteration of a synthesis pathway, such as using sulfonamides to block folic acid synthesis; (4) decreasing permeability of the bacterial cell to the antibiotic; and (5) increasing active efflux of the drugs across the cell surface, pumping out the drugs before damage occurs. This last mechanism is seen in erythromycin resistance in some strains of Streptococcus pyogenes. Moreover, CRE strains employ three different classes of beta-lactamases, all of which can inactivate carbapenem antibiotics. In addition, investigations have determined that colistin resistance is appearing because of the presence of a gene known as mcr-1; this gene is transferred between cells via plasmids (circular extrachromosomal genetic elements), increasing the odds that it will spread throughout the bacterial populations that it encounters. See also: Gene; Plasmid; Streptococcus; Transformation (bacteria)
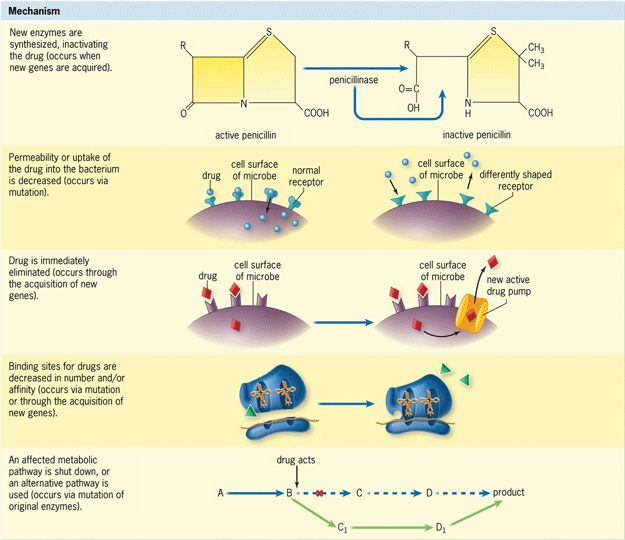
Conclusions
The most significant problem in the development of antibiotic resistance in bacteria is the overuse or misuse of antibiotics by humans. Antibiotics are too easily obtained and prescribed, without sufficient oversight, and they have been used indiscriminately for far too long. For example, in some countries, antibiotics may be purchased over the counter by individuals with no training in medicine, selecting the drug based on a recommendation by a pharmacist, who may or may not be knowledgeable about the patient's condition. Moreover, individuals visit their doctors with viral infections, such as colds or the flu, and are given antibiotics, which have no effect on their actual disease. Finally, antibiotics have been used in agriculture, with almost no control over their use, leading to a particular rise in CRE strains. Therefore, it is important to control the use of antibiotics that are important for human health from being used to treat farm animals. See also: Agricultural science (animal)
Better antibiotic stewardship is imperative, along with better infection control. The only way to stop resistance to antibiotics is through appropriate, guided use of these drugs. Thus, the overall use of antibiotics must be reassessed and reevaluated thoroughly.