Human activity has caused subtle changes in the Earth's atmosphere over the past several decades due to increased emissions of gases such as carbon dioxide (CO2) and methane (CH4). These anthropogenic changes in greenhouse gases have the potential for increasing the temperature in the lower atmosphere due to their ability to absorb infrared radiation. The far-reaching consequences of these changes are now being seen at extremely high altitudes in the outer atmosphere of the Earth, especially in the thermosphere, the high-temperature, near-vacuum region 90–500 km, which is where many satellites are in orbit.
CO2 levels
R. G. Roble and R. E. Dickinson predicted in 1989 that a consequence of increasing CO2 levels would be to decrease the temperature of the upper atmosphere. This is the opposite effect to the response of the lower atmosphere. The reason for this apparent paradox is that CO2 and other multiatom molecules can emit infrared radiation as well as absorb it. In the lower atmosphere, especially in the troposphere (below 15 km), CO2 absorbs radiation coming from the Earth, which excites it to higher vibrational states. Before it can reemit the radiation, it undergoes collisions with other atmospheric gases, transferring the vibrational energy into heat. Any emission that does occur is likely to be reabsorbed by CO2 at other altitudes. But in the stratosphere (15–50 km) and mesosphere (50–90 km) the atmosphere becomes increasingly transparent to infrared radiation as densities decrease, and the radiation-excitation-collision process runs in reverse. Instead of absorbing radiation, CO2 molecules are vibrationally excited by collisions, and then, before additional collisions can remove the vibrational energy, they spontaneously emit in the infrared, which causes radiational cooling of the upper atmosphere.
In the stratosphere, changes in CO2 have a minor effect on temperature because the energy balance is dominated by processes involving ozone (O3). In the mesosphere, CO2 cooling is significant, but long-term changes are difficult to measure. In the thermosphere, one effect of a cooler atmosphere is to cause global density to decrease as the thermosphere cools and contracts. Roble and Dickinson predicted that this could cause a decrease in density of about 40% in the outer atmosphere above ∼300 km for a doubling of CO2 from preindustrial levels, which could happen by 2100. Additional studies by other groups have confirmed these calculations using other models. Changes in density of this magnitude could have significant effects, for example, on the orbits of satellites and space debris, which are affected by drag processes as they pass through the tenuous outer atmosphere. It could also cause changes in the ionosphere, which is the ionized component of the thermosphere, consisting of electrons and positive ions.
Solar cycle
The thermosphere goes through natural, cyclical changes in density driven by the Sun's 11-year activity cycle, heating and expanding at solar maximum, and cooling and contracting at solar minimum. This makes it difficult to detect the smaller and more gradual changes occurring due to increasing anthropogenic emissions. So it may be surprising that during the last several years, three different groups were able to measure thermospheric density changes by observing the effect of atmospheric drag on the trajectories of satellites, some of which have been in orbit since the dawn of the space age. G. M. Keating and coworkers evaluated the long-term orbital decay of five satellites during solar-minimum years and found that density was decreasing at about 5% per decade near 350 km altitude. J. T. Emmert and coworkers derived long-term (secular) trends in upper thermosphere density using 27 near-Earth orbiting objects for all levels of solar activity during the period 1966–2001. Their results indicated that the secular decline ranged 2–5% per decade in the altitude range 200–700 km, with the trend increasing with altitude. F. A. Marcos and coworkers analyzed satellite orbits over the period 1970–2000 and detected an average secular density decrease of 1.7% per decade near 400 km. Since it is expected that temperature change will cause cumulatively larger effects on density at higher altitudes, these observations are in reasonable agreement, except that the initial result from Keating and coworkers indicated somewhat higher trends than either model predictions or other measurements did. To circumvent the problem of the complicating effects of the solar cycle, they confined their study to solar minimum years, which could explain the apparent discrepancy. Emmert and coworkers also observed that the trends were larger at solar minimum than at higher solar activity levels.
Recent work by L. Qian and coworkers employed an extended and updated version of the Roble and Dickinson model to investigate the interaction of anthropogenic change with the solar cycle. They used daily CO2 concentration measurements from the observatory at Mauna Loa, Hawaii, and solar ultraviolet variations, to calculate the secular change of thermosphere neutral density for the last three decades. Results show that the average density decrease at 400 km should be 1.7% per decade, in excellent agreement with Marcos and coworkers observations. They also modeled the expected effect under solar minimum and solar maximum conditions, finding larger trends at solar minimum and smaller change during solar maximum, which could explain some of the observational discrepancies. This is because at solar minimum, CO2 is the primary gas that radiationally cools the thermosphere, but at solar maximum, increases in other minor gases cause CO2 cooling to be less important. The illustration shows results from the 30-year simulations, using the measured CO2 variations and comparing them to a control run with constant CO2. Changes in CH4, O3, H2O, and other minor gases may also affect the upper atmosphere, especially by increasing hydrogen and therefore water vapor in the mesosphere, but their effect on thermospheric temperature and density is nearly an order- of-magnitude less than that of CO2.
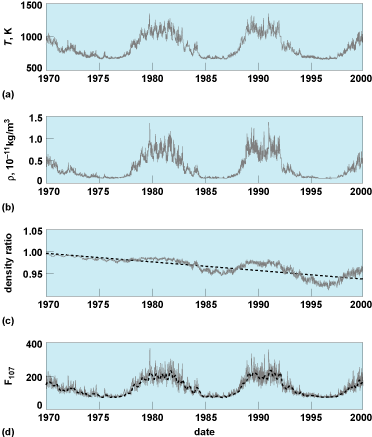
Changes in the ionosphere
These should accompany the neutral atmosphere changes, including slight changes in the height and peak density of ionospheric layers, and decreasing ion temperature. Analysis of long-term ionosonde and radar data has proven challenging, but may also reveal trends. These and other possible effects are reviewed in an article in Science by J. Lastovicka and coworkers. Mesosphere changes are probably occurring but are difficult to prove conclusively because it is not easy to measure temperature accurately and consistently over many decades. But in the thermosphere, where density is a strong function of temperature, theory and observation are in good agreement with regard to density decreases.
Outlook
Continued monitoring and modeling of upper atmospheric change will be important for understanding the complete picture of global change, and for predicting the trajectories of orbiting objects ranging from the smallest fragments to the International Space Station. A beneficial effect could be that it will take less fuel to maintain the orbits of active spacecraft, but defunct satellites, fragments from explosions, and other miscellaneous objects would have even longer orbital lifetimes, which could exacerbate the accumulation of hazardous space debris.
See also: Atmosphere; Climate modeling; Global climate change; Heat balance, terrestrial atmospheric; Ionosphere; Satellite (spacecraft); Solar constant; Thermosphere