Key Concepts
The ability of a planetary atmosphere to inhibit heat loss from the planet's surface, thereby enhancing the surface warming that is produced by the absorption of solar radiation. The greenhouse effect acts like a thermal blanket, limiting heat loss by conduction and convection. In recent decades, the term has also become associated with the issues of global warming and climate change induced by human activity. See also: Atmosphere; Solar radiation
Early studies
The basic concept of the greenhouse effect was first described in 1824 by French mathematician and physicist Joseph Fourier, who studied atmospheric heat flow and pondered how the Earth stays warm enough for plant and animal life to thrive. Fourier realized that much of the thermal radiation emitted by the Earth's surface was being absorbed within the Earth's atmosphere, and that some of this absorbed radiation was being reemitted downward, providing additional warming of the ground surface besides that due to the direct absorption of solar energy (Fig. 1).
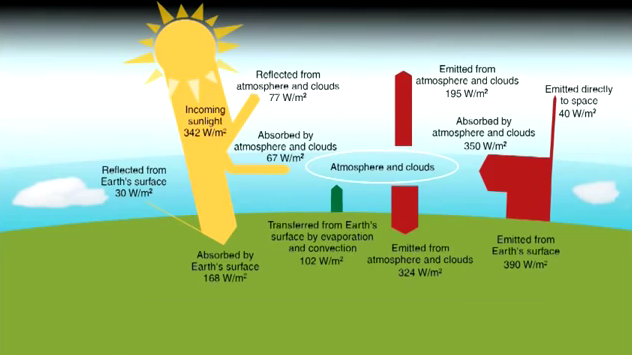
In 1863, Irish physicist John Tyndall provided experimental support for Fourier's greenhouse idea. By means of quantitative spectroscopy, he demonstrated that common atmospheric trace gases, such as water vapor, ozone, and carbon dioxide, are strong absorbers and emitters of thermal radiant energy but are transparent to visible sunlight. In contrast, the principal components of air, nitrogen and oxygen, were found to be radiatively inactive. It was clear to Tyndall that water vapor was the strongest absorber of thermal radiation and therefore highly influential on Earth's surface temperature, but he also speculated in 1861 that a reduction in atmospheric carbon dioxide could induce an ice-age climate.
The Swedish chemist and physicist Svante Arrhenius was the first to develop a quantitative mathematical framework of how the terrestrial greenhouse effect keeps the surface temperature some 33°C (or 60°F) warmer than it otherwise would be. In 1896, Arrhenius showed with heat-balance calculations that reducing atmospheric carbon dioxide by a third would cool global surface temperatures by −3°C (−5.5°F), and that doubling carbon dioxide would warm the tropical latitudes by 5°C (9°F), with somewhat larger warming in polar regions. These results are in remarkably close agreement with current expectations for global climate change in response to carbon dioxide forcing. See also: Spectroscopy
Importance of water vapor
In 1905 the American geologist Thomas Chamberlin described the greenhouse contribution by water vapor as a positive feedback mechanism. Direct sunlight and thermal radiation from greenhouse gases raise surface temperatures and cause more water evaporation, which in turn produces additional heating and further evaporation. When the heat source is taken away, excess water vapor precipitates from the atmosphere, which results in further cooling. Because of this feedback interaction, the temperature change is significantly larger than it would be if the amount of atmospheric water vapor had remained constant. Consequently, there is strong coupling and interaction between the amount of atmospheric water vapor and temperature. Carbon dioxide thus becomes the controlling factor of long-term change in the terrestrial greenhouse effect, but the positive feedback action of water vapor magnifies the resulting change in temperature.
Relative contributions to greenhouse effect
In addition to water vapor, many other feedback interactions operate in the Earth's climate system and impact the sensitivity of the climate response to an applied radiative forcing. Determining the relative strengths of feedback interactions between clouds, aerosols, snow, ice, and vegetation, including the effects of energy exchange between the atmosphere and ocean, is an active research topic. Current best estimates from climate modeling results show that without feedback effects, a doubling of atmospheric carbon dioxide will raise the global surface temperature by 1.2–1.3°C (2.2–2.4°F), but that the net feedback magnification due to water vapor, snow/ice melting, and cloud changes magnifies the no-feedback result by approximately a factor of 3.
Carbon dioxide accounts for approximately 7°C (13°F) of the total 33°C (60°F) terrestrial greenhouse effect, and the other noncondensing greenhouse gases such as ozone, methane, nitrous oxide, and anthropogenic chlorofluorocarbons add another 3–4°C (5–7°F) of greenhouse strength. In this context, the noncondensing greenhouse gases can be thought of as providing the core forcing for the Earth's greenhouse effect. The larger greenhouse contribution by water vapor and clouds may then similarly be considered a feedback response that magnifies the core forcing by a factor of about 3. Carbon dioxide thus becomes the controlling factor of long-term change in the terrestrial greenhouse effect. See also: Climate modeling; Climate modification
Clouds are important contributors to the terrestrial greenhouse effect because they absorb thermal radiation at all wavelengths of the spectrum. The greenhouse efficiency of a greenhouse contributor depends on how strongly it absorbs thermal radiation, and on how weakly the absorbed thermal radiation is reemitted. Yet the emission of thermal radiation has explicit dependence on temperature. Thus a cloud near the ground is a poor contributor to the greenhouse effect because it absorbs and reemits thermal radiation at nearly the same temperature. A cirrus cloud, on the other hand, strongly contributes to the terrestrial greenhouse effect because, being cold itself, it can emit only a small fraction of the absorbed radiation out to space, thus very effectively trapping heat within the atmosphere. See also: Cloud; Heat balance of the Earth
Radiative modeling analyses by G. A. Schmidt and colleagues showed that of the total 33°C (60°F) greenhouse effect, about 50% is contributed by water vapor, 25% by clouds, 20% by carbon dioxide, and the remaining 5% is due to methane, nitrous oxide, ozone, and the chlorofluorocarbon gases. These radiative contributions to the greenhouse effect can then be separated into two groups: the noncondensing greenhouse gases carbon dioxide and the other minor trace gases (Fig. 2), and the condensing species water vapor and clouds.
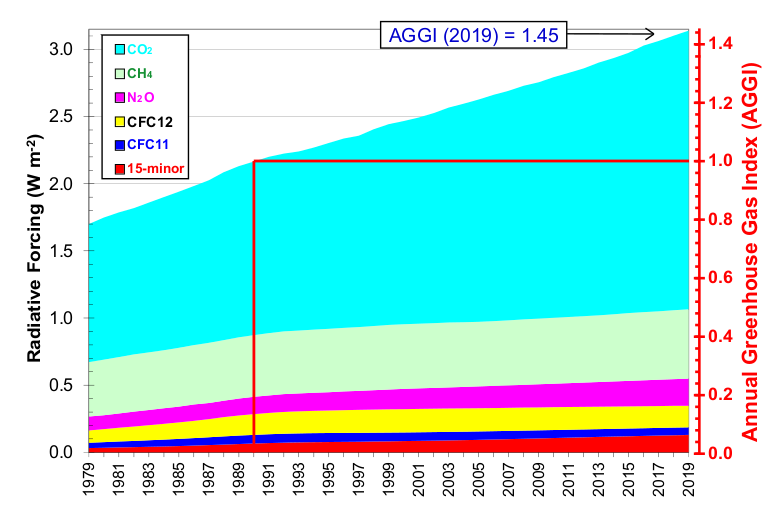
It is not just the greenhouse gases that constitute the greenhouse effect. The atmospheric temperature profile, which emerges from the combined effect of atmospheric dynamics, thermodynamics, and radiative heating and cooling as well as the vertical distribution of the greenhouse gases, determines the full magnitude of the terrestrial greenhouse effect. If radiation were the sole means of energy transport, the terrestrial greenhouse effect would be twice as large, or about 66°C (120°F) warmer than the no- atmosphere reference temperature of –18°C (or about 0°F).
Crucial influence of carbon dioxide
A. A. Lacis and colleagues demonstrated the volatile nature of the terrestrial greenhouse effect in a climate modeling experiment in which all of the noncondensing greenhouse gases were zeroed out. The water vapor quickly condensed and rained out, plunging the modeled climate of Earth into an ice-ball state. This experiment underscores that it is the radiative forcing effects of the noncondensing greenhouse gases (of which carbon dioxide contributes 80%) that provide sustaining support for the terrestrial greenhouse effect. Atmospheric carbon dioxide thus acts as a thermostat to regulate the equilibrium temperature of Earth.
Over geological time, volcanoes have been the principal source of atmospheric carbon dioxide, and the weathering of rocks has been the principal sink. Fifty million years ago, when atmospheric carbon dioxide is believed to have been as high as 2000 parts per million, there was no permanent ice even in the polar regions. About 35 million years ago, when the atmospheric carbon dioxide level had decreased to about 450 parts per million, permanent glaciation began to appear in Antarctica. Ice core measurements covering the past 400,000 years show atmospheric carbon dioxide levels to have varied between about 180 parts per million during the greatest extent of continental ice to about 280 parts per million during the warmer inter-glacial periods.
The current level of atmospheric carbon dioxide stands exceeds 400 parts per million, and it is increasing by about 2 parts per million annually as the direct result of human industrial activity. Given that it is the noncondensing greenhouse gases that provide the sustaining support for the terrestrial greenhouse effect, it follows therefore that the strength of the terrestrial greenhouse effect is increasing as the atmospheric level of carbon dioxide increases. It is only the large ocean heat capacity that keeps delaying the inevitable increase in global temperature that is being built into the climate system.
Given accurate knowledge of the atmospheric concentration of greenhouse gases, their impact on the strength of the atmospheric greenhouse effect can be accurately calculated using the extensive spectroscopic databases that are available now. Inasmuch as the term “greenhouse effect” refers to determining the radiative efficiency of trapping solar heat at the ground surface by the absorption and emission of thermal radiation, a moderate computational effort can provide the answer. Insofar as human activity induces global warming and climate change, it is necessary to incorporate the uncertainties that are associated with atmospheric feedback processes and with atmosphere/ocean dynamical interactions that contribute to greenhouse efficiency. At present, it is necessary to rely on general circulation climate models to determine the feedback contribution (due mostly to clouds and water vapor) to the total atmospheric greenhouse effect, since remote sensing measurements capable of measuring feedback-related water vapor and cloud changes are not currently available. See also: Atmospheric general circulation; Climate modeling; Remote sensing
Anthropogenic warming of the climate
The modern approach to studying Earth's climate and the human impact on climate change began in the 1930s with the work of Guy Callendar, a British engineer who systematically documented the time trend of anthropogenic fossil-fuel use and linked it to the corresponding increase in atmospheric carbon dioxide. From precise spectroscopic measurements of the absorption characteristics of water vapor, carbon dioxide, and other heat-absorbing gases, Callendar linked his estimates of carbon dioxide increase with the 0.4°C (0.7°F) temperature increase recorded in the 50 years prior to 1950. In 1958 Charles Keeling, a research chemist at Scripps Institute in California, began making high-precision measurements of carbon dioxide accumulation in the atmosphere. This ushered in a new era of precise measurement and documentation of the atmospheric increases of carbon dioxide and other greenhouse gases such as methane and nitrous oxide. The new measurement techniques were subsequently applied to measuring the precise gaseous composition of air bubbles trapped in glacial ice, thereby extending knowledge of atmospheric composition over time scales going back 420,000 years based on the Antarctic Vostok ice-core data. Preindustrial concentrations of carbon dioxide were 280 parts per million in 1850. Atmospheric concentrations of carbon dioxide has increased to more than 413 ppm (Fig. 3). In geological context, during the last ice age when the global temperature was about 5°C (9°F) colder, atmospheric carbon dioxide was at levels near 180 ppm.
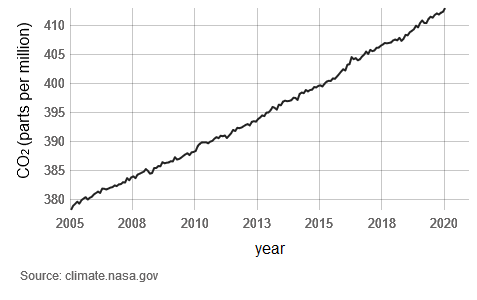
As reviews by the International Panel on Climate Change and thousands of scientific reports have documented, there are good reasons to be concerned about the dangers posed by this new powerful human influence on the greenhouse effect and the Earth’s climate. (1) The projected global warming is much larger than anything previously experienced in human history. (2) The climate response to a globally uniform forcing is not necessarily uniform and may include large regional fluctuations. (3) Extreme events, both droughts and floods, are more likely with the stronger hydrological cycle of a warmer Earth. (4) The inevitable rise in sea level will put low-lying coastal areas at increased risk. (5) Unanticipated changes in global climate could occur if the climate system were to exceed some critical threshold that is beyond the modeling capability of current climate models.
Greenhouse effects on other planets
Of the terrestrial planets, Venus has by far the strongest greenhouse effect. Venus's atmosphere, which is mostly carbon dioxide, has an exceedingly large thermal opacity—100 times greater than that of Earth's atmosphere. As a result, although only about 1% of the incident solar radiation penetrates to the ground, the greenhouse effect on Venus is about 15 times greater than on Earth. The trapped solar radiation on Venus generates a surface temperature of nearly 460°C (860°F), which is hot enough to melt lead and vaporize mercury. (If the surface were simply in thermal equilibrium with the global mean solar energy absorbed by Venus, its temperature would be only −40°C [−40°F]). Venus stands as an example of a runaway greenhouse effect that has rendered the planet inhospitable to life.
In contrast, the thin atmosphere of Mars, with a pressure only one hundredth that of Earth’s atmosphere, can muster only a few degrees (about 5°C or 9°F) of greenhouse warming. On Mercury, which has no tangible atmosphere, there is no greenhouse effect. Mercury's surface temperature is determined solely by local thermal equilibrium with the absorbed sunlight. See also: Mars; Mercury (planet); Venus