Wood is a durable engineering material when used in an appropriate manner, but it is susceptible to biological decay when a log, sawn product, or final product is not stored, handled, or designed properly. Even before the biological decay of wood becomes visually apparent, the decay can cause the wood to become structurally unsound. The progression of decay to that critical state depends heavily on temperature and moisture conditions. As a preventative measure, wood preservatives are chemical treatments applied to wood to protect less-durable wood species from decay. To ensure that these treatments are effective in providing protection, the wood preservation industry typically uses a suite of standard test methods to evaluate the efficacy of wood preservatives to combat wood decay microorganisms. The two standardization agencies in the United States are the American Wood Protection Association (AWPA) and the American Society for Testing Materials (ASTM).
The initial evaluation for determining the feasibility and potential effectiveness of a preservative is based on laboratory tests of small wood blocks, both treated and untreated, which are challenged against wood-rotting fungi. The soil block test (AWPA E10 or ASTM D1413) requires 12–16 weeks of exposure (depending on the test block dimensions) to various decay fungi (for example, brown- or white-rot fungi), and more time is needed for preparing and conditioning the samples before and after the test. The weight loss of treated blocks over this exposure period provides a measure of the minimum amount of preservative necessary for preventing decay under optimum conditions, and it allows the determination of the amount of preservative needed for long-term field testing (aboveground or in-ground field tests). Field tests usually take a minimum of five years of exposure to fully evaluate the effectiveness of preservatives against outdoor hazards. Furthermore, it has been known for years that there can be a significant loss of strength in biologically degraded wood, especially wood under attack from brown-rot fungi, and this strength loss appears long before there is very much weight loss. Accelerated laboratory tests based on strength measurements of early decayed wood are being developed and standardized as early indicators of the performance of wood preservatives. Recently, a standard for predicting aboveground performance has been developed that is based on the compressive strength loss of wood sticks after four weeks in an accelerated fungal exposure (AWPA E22-12). A key for developing accelerated testing methods is to use accurate and quantitative measurements that are important indicators of performance in service conditions. Bending elasticity and compression tests have been used for small specimens, and dynamic modulus of elasticity (MOE) tests have been used for larger size specimens. This article explores the issue of weight loss versus strength loss as a result of decay in the evaluation of wood preservatives.
Decay fungi
Decay fungi are multicellular filamentous microorganisms that use the structural components of wood as food. Figure 1 shows the decay cycle of wood. The fungal spores are spread by the wind, insects, or animals. They germinate on moist, susceptible wood, and the hyphae (mycelial filaments) spread throughout the wood. These hyphae secrete enzymes that attack the wood cells, causing the wood to deteriorate. After serious decay, a new fruiting body may form.
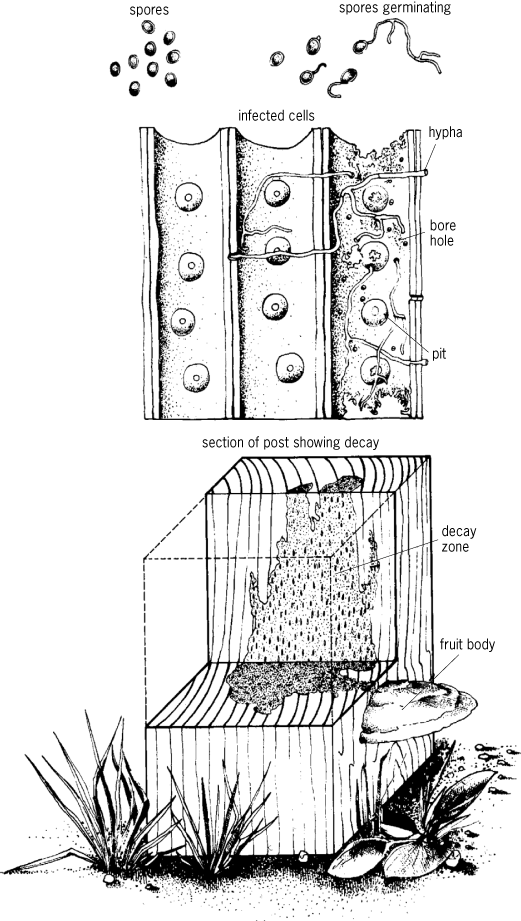
In the early or incipient stage of wood decay, serious strength losses can occur before the decay is even detected. Strength properties related to sudden loads, such as toughness or impact bending, are most sensitive to decay. When affected by incipient decay, unseasoned wood may become discolored, but it is harder to detect on dry wood. The advanced stages of wood decay are easier to detect. Decayed wet wood will break across the grain, whereas sound wood will splinter.
Decay fungi need food (hemicelluloses, cellulose, and lignin), oxygen (air), the proper temperature [10–35°C (50–95°F); optimum: 24–32°C (75–90°F)], and moisture (above the fiber saturation point; approximately 30% moisture content) to grow. Free water must be present (from rain, condensation, or wet ground contact) for the fiber saturation point to be reached and decay to occur. Air-dried wood will usually have no more than 20% moisture content, so decay will not occur. However, there are a few water-conducting fungi that transport water to dry wood and cause a decay called dry rot. When free water is added to wood to attain 25–30% moisture content or higher, decay will occur. Yet, wood can be too wet for decay. If wood is soaked in water, there is not enough air for the fungi to develop.
Brown-rot fungi
Brown-rot fungi decompose the carbohydrates (that is, the cellulose and hemicelluloses) of the wood. The lignin is left intact, making the wood browner in color (hence, the name brown-rot). Brown-rot fungi mainly colonize softwoods, but they can be found on hardwoods as well. Because of the attack on the cellulose, the strength properties of the brown-rot decayed wood decrease quickly, even in the early stages. When extreme decay is attained, the wood becomes a very dark, charred color. After the cross-grain cracking, the wood shrinks, collapses, and finally crumbles. Brown-rot fungi first use a low-molecular-weight system to break down (depolymerize) the cellulose within the cell wall, and then they use specific enzymes (endocellulases) to further decompose the wood.
White-rot fungi
White-rot fungi decompose all of the structural components (that is, the cellulose, hemicelluloses, and lignin) of the wood. As the wood decays, it becomes bleached (in part from the lignin removal) or “white” with black zone lines. White-rot fungi occur mainly on hardwoods, but they can be found on softwoods as well. The degraded wood does not crack across the grain until it is severely degraded. It keeps its outward dimensions, but feels spongy. In general, the strength properties decrease gradually as the decay progresses; however, the toughness can decrease more rapidly. White-rot fungi have a complete cellulase enzyme complex and also the ability to degrade lignin.
Soft-rot fungi
Soft-rot fungi are related to molds. They usually occur in wood that is constantly wet, but they can also appear on surfaces that encounter wet–dry cycling. Typically, the decayed wood is shallow in depth and “soft” when wet, but the un-decayed wood underneath is still firm. Upon drying, the decayed surface is fissured. The wood becomes darker (dull-brown to blue-gray) when decayed by soft-rot fungi. Soft-rot fungi have a system that first frees the lignin in the wood, allowing the cellulase decay enzymes access to the substrate.
Methods for evaluating wood decay in a testing environment
The evaluation of wood decay is complicated because different fungi can attack different wood species in diverse ways. The history of the exposure is also important. In the evaluation of the efficacy of wood preservatives, multiple methods are used to compare the deterioration of preservative-treated wood to untreated wood (as a negative control) and also to wood that has been treated with an established effective preservative (as a positive control). Depending on the test conducted, difficulties can be encountered, including the inability to control for wood moisture content variation during the test, depletion of the wood preservatives during the test (for example, by leaching), biomass weight gain from fungal colonization, and the lack of differentiation between localized and entire specimen decay.
Laboratory decay tests
Laboratory decay tests expose the treated wood to fungi that are known to aggressively degrade less-durable and untreated wood. These tests are based on weight loss or strength loss (or both) and help to determine the threshold treatment level that is needed to prevent the decay of wood that will be in contact with the soil. These tests are typically performed before field testing. Often, metal- or biocide-tolerant strains of wood decay fungi may be used in these tests. Laboratory decay tests are optimized to provide ideal conditions for fungal biodegradation.
Outdoor in-ground field tests
Outdoor in-ground field tests expose treated wood stakes and untreated controls to various natural organisms under severe conditions. Wood stakes are placed in the soil and rated visually over an extended period of time. Usually two different sites are chosen with different moisture and temperature climates, soil properties, and types of organisms present.
Outdoor aboveground field tests
Outdoor aboveground field tests expose treated wood and untreated controls to the weather (usually in an area with a warm, wet climate), and they are visually rated over an extended period of time. The exposures are usually not as severe as in-ground field stake tests. The wood samples are installed and designed to trap moisture and create ideal conditions for aboveground decay.
Strength tests
Strength tests are a way of comparing the mechanical properties of wood, including treated versus untreated wood exposed to similar conditions. Changes in the mechanical properties can be indications that the wood has been damaged by biological decay or even from the chemical treatment or process. AWPA Standard E22-12 is an accelerated laboratory method for testing the efficacy of preservatives against decay fungi using compressive strength, which may be used as an indicator of aboveground performance.
Bending, compression, hardness, and the torsional properties of wood at various stages of fungal decay have been measured. Static bending has been shown to be a viable method of measuring decay in the laboratory. Tests to determine the MOE with static bending require specialized equipment and controlled conditions, and these tests are usually done in a laboratory. A dynamic methodology called dynamic MOE (MOEdyn) is another way to determine the MOE and is highly correlated with the static MOE. It was initially developed on the basis of an ultrasonic pulse excitation methodology; however, a more recent MOEdyn method has been developed using resonant vibration excitation technology. With this type of nondestructive measurement, there are fewer sample dimension and configuration restrictions, and on-site measurements can be performed with the equipment. Handheld spring-loaded plunger devices have also been used to correlate wood hardness with the extent of decay. A strong correlation exists at higher weight losses, but the measurements are affected by grain orientation and moisture content, making it difficult to detect the early stages of decay.
Other methods
Methods to detect the presence of fungal antigens have been developed (immuno-diagnostics) and are very sensitive to detecting the incipient stages of decay, but they do not provide information on the progressive development of the decay. However, genetic profiles of microbial communities in wood decay environments are being developed. Establishing relationships between the progression of these measurements and strength properties could provide quicker methods for incipient and early wood decay evaluation.
Near-infrared (NIR) spectroscopy has been used to detect and quantify the chemical biodeterioration of wood. The coupling of NIR spectroscopy with multi-variate regression or principal components analysis has allowed good correlations to be made between NIR measurements and both mass loss and compressive strength loss of brown-rotted wood. However, more research is needed to determine if this method is capable of detecting the early stages of decay and other types of fungi.
Effect of decay on the strength of wood
Decay initially affects toughness, or the ability of wood to withstand impacts. This is generally followed by reductions in strength values related to static bending. Eventually, all strength properties are seriously reduced. Strength losses during the early stages of decay can be considerable, depending to a great extent on the fungi involved and to a lesser extent on the type of wood undergoing the decay. In laboratory tests, the losses in toughness ranged from 6% to more than 50% by the time that a 1% weight loss had occurred in the wood as a result of fungal attack. Further, the weight loss of wood in the range of 5–10% equates to a reduction of the mechanical properties in the range of 20–80%. At weight losses of 10% or less, the decay is detectable only microscopically. A wood that shows visible signs of decay most likely has greatly reduced strength values. There is no method known to estimate the amount of strength loss from just visually inspecting the decayed wood.
Of the three main components of wood, that is, cellulose, hemicelluloses, and lignin, the initial losses in strength from early brown-rot decay are the result of the attack on the hemicelluloses and then the cellulose. At low weight losses in the decayed wood, there can be significant reductions in the degree of polymerization of the holocellulose (the total polysaccharide fraction of the wood); for example, at only 10% weight loss of sweetgum decayed by a brown-rot fungus, the degree of polymerization of the holocellulose dropped from 1500 to 300. The wood's mechanical properties decrease when the side chains of the hemicelluloses (such as arabinose and galactose) are degraded enzymatically by chemical reactions, including hydrolysis, dehydration, and oxidation. The initial strength loss of 5–20% is related to the initial degradation of the hemicelluloses, followed by the degradation of the main-chain backbones of the hemicelluloses. After 40–60% strength reduction, the glucose and lignin are degraded. This helps explain the shortcomings of using mass loss to detect the early stages of decay.
Figure 2 shows the effect of decay by a brown-rot fungus (Gloeophyllum trabeum) on the weight loss and mechanical properties of wood. It shows that the loss in bending strength occurs before any measurable weight loss. At a 10% weight loss, there is an approximately 40% loss of strength (modulus of rupture, MOR) and a 70–80% loss in energy properties, such as the work to the maximum load (WML).
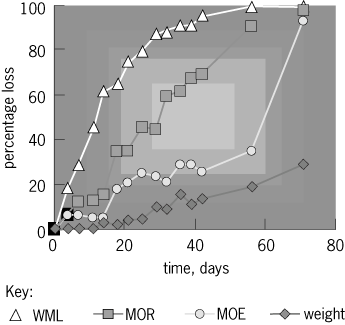
Figure 3 shows a comparison of the loss of the carbohydrate components with the loss in bending strength (MOR) as the result of the decay caused by a brown-rot fungus (Gloeophyllum trabeum). The chemical composition is directly related to the strength loss (MOR).
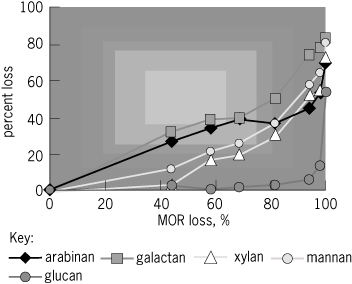
The table shows the losses in MOE and maximum compressive strength (MCS) in wood exposed to a brown-rot fungus (Postia placenta) in the standard ASTM soil block and in-ground tests. The data show that there are critical losses in MOE and strength measurements in both types of tests, even when there are low levels of weight loss.
Wood blocks |
Wood stakes | |||||
---|---|---|---|---|---|---|
Time, |
Weight loss, |
MOE reduction, |
MCS reduction, |
Weight loss, |
MOE reduction, |
MOR reduction, |
weeks |
% |
% |
% |
% |
% |
% |
1 |
0 |
3 |
6 |
0 |
5 |
2 |
2 |
3 |
13 |
21 |
0 |
8 |
2 |
3 |
9 |
22 |
37 |
1 |
7 |
8 |
4 |
18 |
34 |
53 |
3 |
9 |
19 |
5 |
26 |
53 |
71 |
7 |
12 |
22 |
Note: The brown-rot fungus Postia placenta was used in the tests. MOE, modulus of elasticity; MCS, maximum compressive strength; MOR, modulus of rupture. [Table adapted from C. A. Clausen and S. N. Kartal, Accelerated detection of brown-rot decay: Comparison of soil block test, chemical analysis, mechanical properties and immunodetection, For. Prod. J., 53(11/12):90–94, 2003]
In service, the heterogeneous nature by which decay fungi consume and deconstruct wood makes the field evaluation of strength properties difficult. This known, rapid loss in strength properties, which can occur prior to visual detection or weight loss, combined with the fact that wood is susceptible to other hazards if it is not prepared, stored, or treated properly, means that wood suspected of deterioration should be inspected by a professional and repaired or replaced if necessary. Wood handled and treated in a proactive manner to prevent attack by decay and other hazards reduces the risk of strength losses and makes wood a cost-effective building material.
See also: Biodegradation; Cellulose; Enzyme; Fungal ecology; Fungi; Hemicellulose; Lignin; Lignin-degrading fungi; Strength of materials; Wood anatomy; Wood degradation; Wood engineering design; Wood products; Wood properties