Key Concepts
Any of the organic compounds possessing one or more basic amino groups and one or more acidic carboxyl groups. Of the more than 80 amino acids that have been found in living organisms, approximately 20 serve as the building blocks for proteins. These 20 amino acids are typically divided into essential (indispensable) and nonessential (dispensable) groups, which can vary for different organisms (the essential and nonessential amino acids for humans are listed in the table). In addition, a number of nonessential amino acids can be considered as essential under certain conditions. See also: Amino acid metabolism; Protein
Essential amino acids | Nonessential amino acids |
---|---|
Histidine | Alanine |
Isoleucine | Asparagine |
Leucine | Aspartic acid |
Lysine | Glutamic acid |
Methionine | Serine |
Phenylalanine | Arginine* |
Threonine | Cysteine* |
Tryptophan | Glutamine* |
Valine | Glycine* |
Proline* | |
Tyrosine* |
†Under certain conditions, some nonessential amino acids can be considered as essential. These amino acids are marked with an asterisk.
All the amino acids of proteins, and most of the others that occur naturally, are α-amino acids, meaning that an amino group (—NH2) and a carboxyl group (—COOH) are attached to the same carbon atom. This carbon (the α carbon, being adjacent to the carboxyl group) also carries a hydrogen atom; its fourth valence is satisfied by any of a wide variety of substituent groups, represented by the letter R in the structural formula shown in Fig. 1. See also: Biochemistry; Carbon; Hydrogen
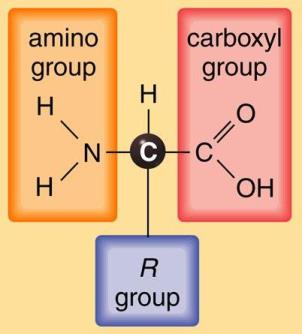
In the simplest amino acid, that is, glycine, R is a hydrogen atom. In all other amino acids, R is an organic radical; for example, it is a methyl group (—CH3) in alanine, whereas it is an aliphatic chain terminating in a second carboxyl group (—CH2—CH—COOH) in glutamic acid. Chemically, the amino acids can be categorized according to the nature of R (Fig. 2).
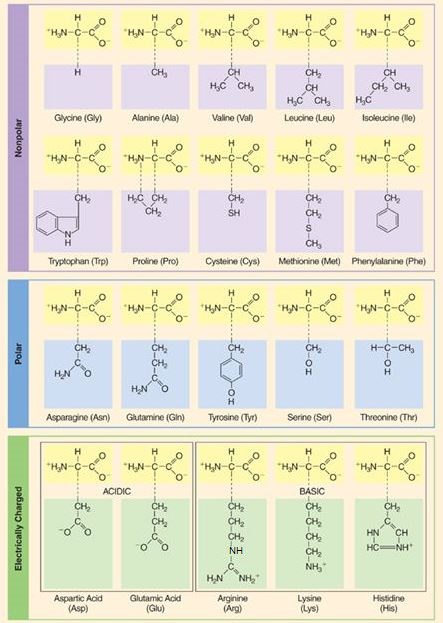
Occurrence of conjugated amino acids
Amino acids occur in living tissues principally in the conjugated form (which is created by the union of two or more amino acids or the union of one amino acid with other chemical groups attached by covalent bonds or weak interactions). Most conjugated amino acids are peptides, in which the amino group of one amino acid is linked to the carboxyl group of another. This type of linkage is known as a peptide bond; a molecule of water is split out when a peptide bond is formed (Fig. 3), and a molecule of water must be added when a peptide bond is broken. See also: Peptide
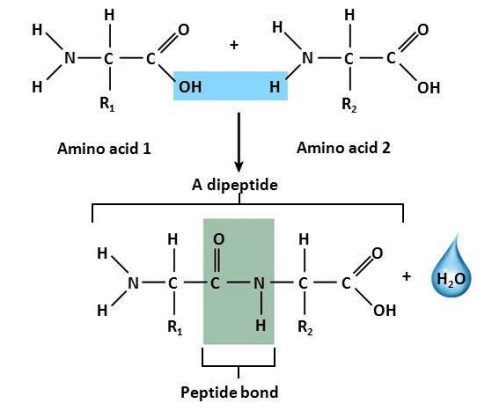
Amino acids are capable of linking together to form chains of various lengths, called polypeptides. Proteins are polypeptides ranging in size from about 50 to many thousand amino acid residues (Fig. 4).
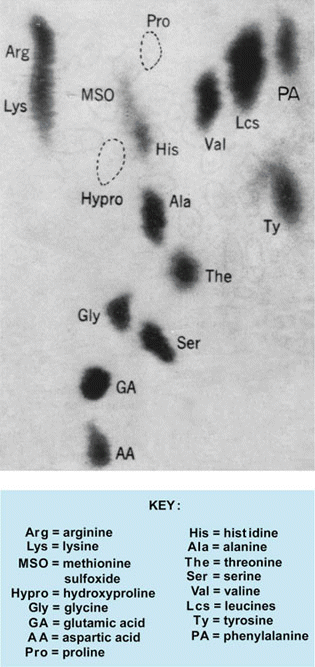
Although most of the conjugated amino acids in nature are proteins, numerous smaller conjugates occur naturally, and many of these have important biological activity. The line between large peptides and small proteins is difficult to draw, with insulin (molecular weight = 7000; 50 amino acids) usually being considered a small protein and adrenocorticotropic hormone (molecular weight = 5000; 39 amino acids) being considered a large peptide. In addition to their role as hormones, peptides often occur in coenzymes (for example, folic acid and glutathione), bacterial capsules (for example, the polyglutamic acid capsule that contributes to the pathogenicity of Bacillus anthracis), fungal toxins (for example, the tomato wilt toxin of Fusarium and the toxins of the poisonous mushroom Amanita phalloides), and antibiotics (for example, chloramphenicol, penicillin, bacitracin, and polymixins). See also: Antibiotic; Coenzyme; Hormone; Toxin
Occurrence of free amino acids
Free amino acids are found in living cells, as well as in the body fluids of higher animals, in amounts that vary according to the tissue and to the amino acid. The amino acids that play key roles in the incorporation and transfer of ammonia, such as glutamic acid, aspartic acid, and their amides, are often present in relatively high amounts, but the concentrations of the other amino acids of proteins are extremely low, ranging from a fraction of a milligram to several milligrams per 100 gram wet weight of tissue. The presence of free amino acids in only trace amounts points to the existence of extraordinarily efficient regulation mechanisms. Each amino acid is ordinarily synthesized at precisely the rate needed for protein synthesis. The regulation mechanism has been found most often to be one of feedback control; each amino acid acts as an inhibitor of its own biosynthesis. If any amino acid is formed in excess of that required for protein synthesis, the biosynthesis of that amino acid is slowed down until the excess has been used. See also: Biosynthesis
In addition to the amino acids of proteins, a variety of other free amino acids occurs naturally. Some of these are metabolic products of the amino acids of proteins; for example, γ-aminobutyric acid occurs as the decarboxylation product of glutamic acid. Others, including homoserine and ornithine, are biosynthetic precursors of the amino acids of proteins. However, the origin and role of many unusual free amino acids are not yet known.
General properties
The amino acids are characterized physically by the following factors: (1) the pK1, or the dissociation constant of the various titratable groups; (2) the isoelectric point, or pH at which a dipolar ion does not migrate in an electric field; (3) the optical rotation, or the rotation imparted to a beam of plane-polarized light (frequently the D line of the sodium spectrum) passing through 1 decimeter of a solution of 100 grams in 100 milliliters; and (4) solubility. See also: Ionic equilibrium; Isoelectric point; Optical activity; pH
Because all of the amino acids, except glycine, possess a center of asymmetry at the α carbon atom, they can exist in either of two optically active, mirror-image forms or enantiomorphs. All of the common amino acids of proteins appear to have the same configuration about the α carbon; this configuration is symbolized by the prefix l-. The opposite, generally unnatural, form is given the prefix d-. Some amino acids, such as isoleucine, threonine, and hydroxyproline, have a second center of asymmetry and can exist in four stereoisomeric forms. The prefix allo- is used to indicate one of the two alternative configurations at the second asymmetric center; thus, isoleucine, for example, can exist in the l, l-allo, d, and d-allo forms. See also: Stereochemistry
At ordinary temperatures, the amino acids are white crystalline solids; when heated to high temperatures, they decompose rather than melt. They are stable in aqueous solution, and with few exceptions can be heated as high as 120°C (248°F) for short periods without decomposition, even in acid or alkaline solution. Thus, the hydrolysis of proteins can be carried out under such conditions with the complete recovery of most of the constituent free amino acids.
Biosynthesis
Because amino acids, as precursors of proteins, are essential to all organisms, all cells must be able to synthesize those amino acids that they cannot obtain from their environment. The selective advantage of being able rapidly to shift from endogenous to exogenous sources of these compounds has led to the evolution in bacteria and many other organisms of very complex and precise methods of adjusting the rate of synthesis to the available level of the compound.
An immediately effective control is that of feedback inhibition. The biosynthesis of amino acids is relatively complicated and usually requires at least three enzymatic steps. In most cases so far examined, the amino acid end product of the biosynthetic pathway inhibits the first enzyme to catalyze a reaction that is specific to the biosynthesis of that amino acid. This inhibition is extremely specific; the enzymes involved have special sites for binding the inhibitor. This inhibition functions to shut off the pathway in the presence of transient high levels of the product, thus saving both carbon and energy for other biosynthetic reactions. When the level of the product decreases, the pathway begins to function once more. See also: Enzyme; Enzyme inhibition
The metabolic pathways by which amino acids are synthesized generally are found to be the same in all living cells, whether microbial or animal. Biosynthetic mechanisms thus appear to have developed soon after the origin of life and to have remained unchanged through the divergent evolution of modern organisms. The major exception is lysine, which is formed from aspartic acid via diaminopimelic acid in bacteria, but from α-ketoglutaric acid in fungi. Indeed, the occurrence of diaminopimelic acid as a precursor of lysine, or as a constituent of proteins, or both, is a major taxonomic property of bacteria and the related cyanobacteria (formerly referred to as blue-green algae). See also: Bacteria; Cyanobacteria; Fungi
Formation and transfer of amino groups
Biosynthetic pathway diagrams reveal only one quantitatively important reaction by which organic nitrogen enters the amino groups of amino acids: the reductive amination of α-ketoglutaric acid to glutamic acid by the enzyme glutamic acid dehydrogenase. All other amino acids are formed either by transamination (transfer of an amino group, ultimately from glutamic acid) or by a modification of an existing amino acid. An example of the former is the formation of valine by transfer of the amino group from glutamic acid to α-ketoisovaleric acid; an example of the latter is the reduction and cyclization of glutamic acid to form proline. See also: Nitrogen; Transamination
Amino acids in nutrition
The nutritional requirements for the amino acids of proteins can vary from zero, in the case of an organism that synthesizes them all, to the complete list, in the case of an organism in which all the biosynthetic pathways are blocked. There are 8 to 10 amino acids required by certain mammals; most plants synthesize all of their amino acids, whereas microorganisms vary from types that synthesize all of them to others (such as certain lactic acid bacteria) that require the intake of as many as 18 different amino acids. See also: Nutrition
It seems likely that, when life originated, amino acids were taken from the rich organic medium that the oceans then offered, and biosynthetic abilities evolved only slowly as the supply of exogenous materials became depleted. A stage must have eventually been reached, however, at which all the amino acids were being synthesized metabolically, and none was required nutritionally. As evolution progressed, food chains developed, and some forms of life became adapted to obtain many of their organic nutrients at the expense of other living forms, either directly or indirectly. In these dependent types, mutations had occurred, causing the loss of specific biosynthetic enzymes and hence the gain of nutritional requirements. It is easy to duplicate this process in the laboratory; for example, a microorganism with full biosynthetic ability can be induced to undergo random mutations, and selective methods can then be used to isolate mutants that require amino acids, vitamins, or other normal metabolites. In every case, it is found that a given mutation deprives the cell of a single biosynthetic enzyme, blocking the reaction which that enzyme catalyzes and thus the entire pathway of which that reaction is a part. See also: Food web; Mutation; Prebiotic organic synthesis
In summary, the nutrition of many organisms must include the provision of growth factors, which are defined as organic compounds that the organism requires for its growth, but which it cannot synthesize. Growth factor requirements reflect the heritable loss of biosynthetic enzymes as the result of gene mutations. Amino acids are typical growth factors for many organisms. See also: Growth factor