Reversal in the polarity of Earth’s magnetic field. That is, at different times in Earth's past, a compass would have pointed south instead of north. The Earth's magnetic field has reversed polarity hundreds of times. Recognition that the geomagnetic field has repeatedly reversed polarity played a key role in the revolution that transformed the geological sciences in the 1960s—the acceptance of the theory of plate tectonics. It is generally accepted that the geomagnetic field is generated by motion of electrically conducting molten metal in Earth's outer core. However, the mechanism by which the field decays and reverses polarity remains one of the great unknowns in geophysics. See also: Geomagnetism; Plate tectonics
The last magnetic field reversal occurred long before humans were aware of the geomagnetic field (780,000 years ago; Fig. 1), so it is necessary to study geological records to understand the process by which the field reverses. The ability of rocks to act as fossilized compasses, which record a permanent “memory” of Earth's magnetic field at the time of rock formation, makes them suitable for detailed studies of ancient geomagnetic field behavior. See also: Paleomagnetism; Rock magnetism
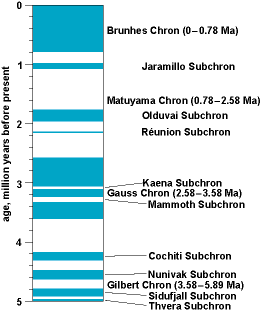
In order to understand the mechanism of field reversal, it is necessary to develop a realistic view of the geometry of the field during polarity transitions. The interval of time required for the field to switch polarity is between about 5000 and 10,000 years. In a typical sedimentary rock unit, a polarity transition is recorded across a thin interval of strata, generally less than a meter thick. Many detailed studies have been undertaken from closely spaced samples through such intervals. During the early 1990s, a compilation of existing records indicated a distinct pattern in the way the field behaves during reversals. In particular, if the position of the magnetic pole was plotted as it progressed through a reversal (Fig. 2), it seemed that a large proportion of the poles tracked across a longitudinal band through the Americas. A second set of poles followed a path on the other side of the Earth (180° from the first) through western Australia and east Asia. This compilation suggested that the transitional field was dominantly dipolar and that biased field configurations may have persisted for several reversals during the last 10 million years. The time constants of convective motion in Earth's fluid outer core are a great deal shorter than in the solid overlying mantle. The persistence of these patterns over such long time intervals therefore suggested that fluid motion in the core is strongly affected by Earth's mantle and that the proposed bias may reflect long-term characteristics of deep-Earth dynamics. The coincidence in the location of transitional geomagnetic poles and other geophysical observations, such as anomalously cool lower-mantle temperatures, fluid flow patterns at the top of Earth's outer core, and significant features in the present geomagnetic field, excited a great deal of interest. As a result, the nature of transitional geomagnetic field behavior became one of the most hotly debated issues in geophysics in the 1990s. See also: Earth, convection in
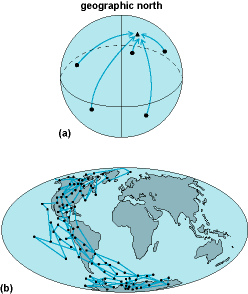
When observations concerning transitional geomagnetic field behavior were subjected to more rigorous statistical analysis, it was concluded that it is premature to accept the hypothesis of lower-mantle control of the geodynamo. Despite the compilation of many polarity transition records, there is an uneven distribution of sites around the world from which polarity transition records are available. A much wider distribution of sites is required for adequate testing of current hypotheses concerning transitional field behavior. In addition, there is considerable concern about the ability of some geological materials, particularly sediments, to record the geomagnetic field during periods when the field is extremely weak (such as during a reversal when the field decays to about one-tenth of its nontransitional value). Thus, rigorous methods must also be developed for assessing the reliability of the records used to make inferences about transitional field behavior. See also: Geodynamo
Major advances in understanding geomagnetic field behavior have recently been made using numerical models that require thousands of hours of super-computer processing. The models provide fairly realistic simulations for how the geomagnetic field is generated, and a polarity reversal has been simulated. This simulation displays many features in common with paleomagnetic records of reversals, but much more work is needed to get a more realistic match. Paleomagnetic observations of polarity transitions are still required from widely distributed sites around the world. Debate concerning geomagnetic field behavior during polarity transitions is therefore far from settled and is likely to rage for some time to come.