Key Concepts
A term that traditionally refers to the substance of which all bodies consist. In everyday usage, matter refers to the ubiquitous materials made of atoms, which in turn are made up by subatomic particles. In modern physics and chemistry, matter refers to any substance that possesses mass and volume. On a granular level, matter is thus composed of elementary particles with mass, namely quarks and leptons, which interact via boson particles and are all described by the standard model of particle physics. Matter does not include massless particles such as photons, and nor does it encompass energy, which is interconvertible with matter. Matter occurs in various states, or phases, most familiarly as a solid, liquid, or a gas, but also as plasma, Bose-Einstein condensate, and numerous other states. See also: Atom; Bose-Einstein condensation; Elementary particle; Energy; Gas; Liquid; Phase transition; Plasma (physics); Standard model
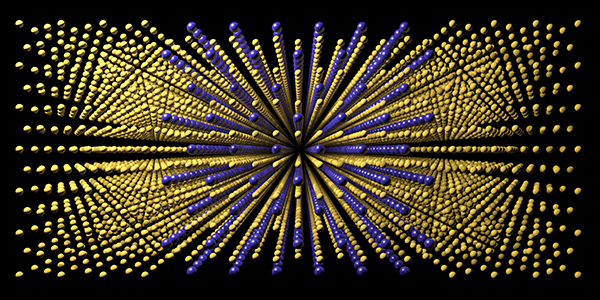
History
In Aristotelian physics, each type (species) of material body had a distinct “essential form.” Early modern scientists, however, asserted that there is one universal type of matter. For English physicist and mathematician Isaac Newton, this matter consisted of “solid, massy, hard, impenetrable, movable,” ultimate particles (“atoms”), which were discrete, localized, indivisible bodies. Modern analyses distinguish two types of mass in classical (Newtonian) matter: inertial mass, by which matter retains its state of rest or uniform rectilinear motion in the absence of external forces; and gravitational mass, by which a body exerts forces of attraction on other bodies and by which it reacts to those forces. Expressed in appropriate units, these two properties are numerically equal—a purely experimental fact, unexplained by theory. German-born U.S. theoretical physicist Albert Einstein made the equality of inertial and gravitational mass a fundamental principle (principle of equivalence), as one of the two postulates of the theory of general relativity. In the equation E = mc2 (where c is the velocity of light), Einstein recognized the equivalence (interconvertibility) of mass (m) and energy (E), which had been distinguished in classical theories. See also: Gravity; Inertia; Mass; Relativity; Weight
Departures from classical thinking about matter emerged with the rise of quantum mechanics in the early decades of the twentieth century. Matter in classical mechanics is closely identified with mass; in quantum mechanics, mass is only one among many properties (quantum numbers) that a particle can have, for example, electric charge, spin, and parity. The nearest quantum-mechanical analogs of traditional matter are fermions, having half-integral values of spin. Forces are mediated by exchange of bosons, particles having integral spins (such as photons). Fermions correspond to classical matter in exhibiting impenetrability (a consequence of the exclusion principle), but the correspondence is only rough. For example, fermions can also be exchanged in interactions (a photon and an electron can exchange an electron), and they also exhibit wavelike behavior. States of classical matter-particles were given by their positions and momenta, but in quantum mechanics it is impossible to assign simultaneous precise positions and momenta to particles. Finally, two or more particles in quantum theory can become “entangled,” so that, in the case of two particles that become entangled and separate by a very great distance, an operation on one particle will be immediately responded to by a corresponding change in the other. This “nonlocality” violates traditional notions of what a piece of matter must be, which led Einstein to refer to quantum mechanics as objectionable in implying a “spooky action at a distance.” See also: Exclusion principle; Nonrelativistic quantum theory; Quantum electrodynamics; Quantum mechanics; Quantum statistics
Matter in the universe
Beginning in the 1920s, astronomers accumulated a vast amount of evidence leading to the conclusion that the density of matter in the universe was much larger than that of visible matter. Important steps in this direction were taken in 1933, when Swiss astronomer and astrophysicist Fritz Zwicky found that the amount of visible matter in a number of galaxy clusters was far too small to hold the galaxies in the cluster, and in 1983, when U.S. astronomer Vera Rubin and colleagues found that the rotation curves of individual spiral galaxies implied that the galaxies must be surrounded by a sphere of unseen matter, ten times the amount in the visible galaxy itself. It began to appear that the visible matter might constitute only a few percent of the amount of matter required, in the simplest theories, to equal the critical density at which the geometry of the universe would be Euclidean (“flat”), and at which the expansion rate of the universe would gradually slow down, asymptotically approaching zero. Some scientists accepted the implication that the universe was “open,” with a hyperbolic geometry and continued expansion forever at a rate that did not approach zero.
Others proposed that missing matter, undetectable by current techniques, might equal the critical density, and many proposals were made regarding the nature of the missing matter. The motivation for seeking the missing matter became stronger with the proposal of the theory of inflation in the early 1980s, which required a critical density. However, visible matter is baryonic, consisting almost entirely by mass of protons and neutrons. With the total density of baryonic matter so low, the missing nonbaryonic dark matter would have to be some kind of exotic particle. Candidates for this status included axions and the lightest (nondecaying) supersymmetric particles, photinos or perhaps winos. None of these, nor many other candidates, have been observed. See also: Dark matter; Supersymmetry; Weakly interacting massive particle (WIMP)
It was long realized that such exotic matter was still not enough for closure (critical density), however, and debates arose regarding the nature of the dark energy that was needed to provide the remainder. One popular proposal was that of quintessence, a negative-pressure energy field, perhaps created by particles that had not been suggested in current hypotheses. However, while quintessence theories have not been entirely ruled out, they have been replaced by a different approach. In 1998, results from studies of distant supernovae indicated strongly that the universe is not merely expanding: the rate of expansion is accelerating. It was found that this acceleration has been going on for approximately five billion years. This new view thus suggested that some kind of dark antigravitational energy was at work, a product of the vacuum rather than, as with quintessence views, some unknown form of quasimatter. It thus resembles the cosmological constant that Einstein had proposed as a term in his equations designed to preserve the universe from the possibility that it might expand or contract. He abandoned this term after the discovery of the expansion of the universe. See also: Accelerating universe; Cosmological constant; Dark energy
Measurements of the cosmic microwave background by observatories including the Wilkinson Microwave Anisotropy Probe (WMAP), the Herschel Space Observatory, and ground-based telescopes have converged on describing the distribution of the universe's composition as follows: 4% of the matter is baryonic, 23% is dark nonbaryonic matter, and 73% is dark energy. Investigations continue through today on further describing the properties of matter, as well as identifying the constituents of dark matter and the nature of dark energy. See also: Cosmic background radiation; Cosmology; Herschel Space Observatory; Universe; Wilkinson Microwave Anisotropy Probe
Antimatter
Matter also exists in a form known as antimatter, consisting of so-called antiparticles that have the opposite charge and differences in quantum numbers from "ordinary" matter. Antimatter is created naturally by certain astrophysical processes as well as in certain kinds of radioactive decay. Antimatter can be generated artificially in small quantities by particle accelerators. See also: Antimatter; Electric charge; Quantum numbers; Particle accelerator; Radioactivity