Key Concepts
An increase in the expansion rate of the universe, attributed to dark energy. In 1998, astronomers presented evidence that the universe's expansion is not slowing down, as would be expected due to the gravity from the abundances of matter strewn throughout the cosmos. Instead, the observations of distant, exploded stars revealed that cosmic expansion is accelerating (Fig. 1). Since this initial discovery, multiple other lines of evidence, including observations of the afterglow of the big bang, known as the cosmic microwave background, and in the clustering of galaxies over cosmic history have made the case for this surprising result more secure. See also: Big bang theory; Cosmic background radiation; Cosmology; Matter (physics); Physics; Universe
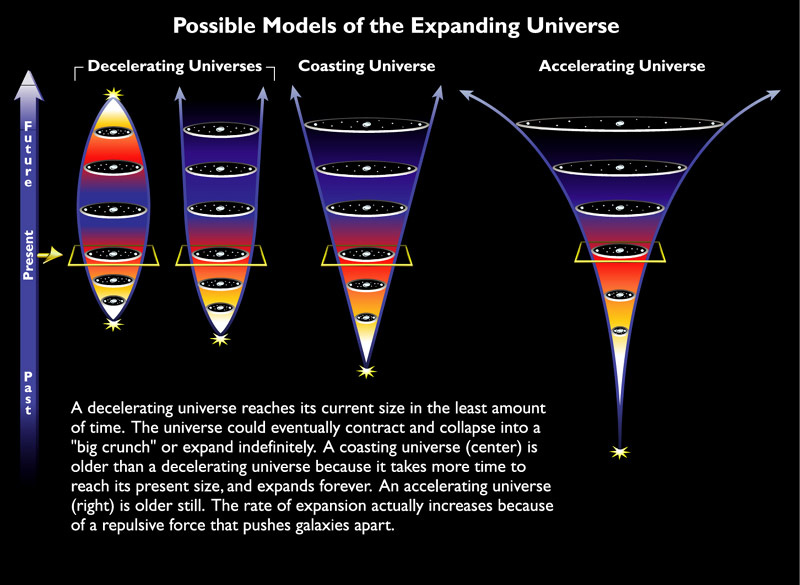
Most cosmologists attribute the acceleration to a mysterious "dark energy," which does not yet have a secure foundation in fundamental physics. Overall, dark energy is estimated to make up about two-thirds of the energy density of the universe. Elucidating its true nature and role in the accelerating universe is one of the biggest challenges in modern science. See also: Astronomy; Dark energy
Discovery of an expanding universe
Before the 1920s, astronomers thought that the Milky Way Galaxy, of which our Sun is but one of an estimated 300 billion stars, constituted the entire universe. U.S. astronomer Edwin Hubble demonstrated this was not the case. Working at the Mount Wilson Observatory in Los Angeles County, Hubble observed luminous objects with an evident spiral structure, then known as spiral nebula. Within them, Hubble measured the apparent brightness of stars, called Cepheid variables, whose intrinsic brightnesses are well-known; the fainter such stars appear, the more distant they must be. In so doing, Hubble demonstrated that the distances to even the nearest spiral nebulae were a few million light-years, far larger than the established size of the Milky Way. Astronomers began to understand these objects as independent galaxies, each equivalent to the Milky Way. See also: Cepheid variables; Galaxy; Light-year; Milky Way Galaxy
Hubble also measured the spectra of galaxies, which revealed their spectrum lines as being shifted toward redder colors. This so-called redshift, akin to a Doppler effect for sound, indicated the galaxies were receding away from us, the observers. By combining the measurements of distance and of recession, Hubble discovered that the more distant galaxies are moving away from us more rapidly. These results, published in 1929, first established that the universe is expanding. See also: Doppler effect; Hubble constant; Redshift; Spectrum
Discovery of an accelerating universe
In the 1990s, astronomers learned to use thermonuclear supernova explosions as distance-measuring tools. Through instruments such as the Hubble Space Telescope, these supernovae are bright enough to be seen more than ten billion light-years away. The measurements with the supernovae rely on the same principle that the telescope's namesake, Edwin Hubble, used in observing Cepheid variables. The supernovae, however, are millions of times brighter, thus allowing for similar measurements over a span of distance (and cosmic time) that is thousands of times larger. See also: Hubble Space Telescope; Supernova
In 1998 and 1999, two research groups published the first papers indicating the accelerating expansion of the universe. The first group, called the High-Z Supernova Search Team, was led by U.S.-born Australian astronomer Brian Schmidt of the Australian National University; the second group, the Supernova Cosmology Project Team, was led by U.S. physicist and cosmologist Saul Perlmutter of the University of California at Berkeley. (The author of this article, Kirshner, was a member of the High-Z Supernova Search Team, and 11 of its 19 members were either students or coworkers of Kirshner's at Harvard University.) Schmidt, Perlmutter, and U.S. astrophysicist Adam Riess of Johns Hopkins University, who served as lead author of the High-Z Supernova Search Team's paper, were awarded the 2011 Nobel Prize in Physics for the discovery. See also: Nobel Prizes for 2011
Specifically, the astronomers observed a kind of supernova designated type Ia (Fig. 2). Type Ia supernovae can be recognized by their spectra. They are found in all types of galaxies and are thought to result from a sudden thermonuclear burning wave that rips through a white-dwarf star as it approaches its maximum possible mass. This process changes the carbon and oxygen of the stellar interior into radioactive nickel, destroying the star. The nickel's subsequent radioactive decay into cobalt and iron releases energy gradually as the star flies apart. For about a month, a single type Ia supernova shines several billion times as brightly as the Sun. This property makes type Ia supernovae visible over very large distances. In addition to being bright, type Ia supernova also have relatively uniform intrinsic luminosities, which enables comparisons between them and thus distant estimations, as with Cepheid variables. Accordingly, type Ia supernovae are the one of the best distance-measuring tools for surveying the distant universe. The effect of cosmic acceleration is to make distant type Ia supernovae appear a little fainter than they would otherwise be. The size of this effect is about a 20% reduction in brightness. See also: Light curves; Stellar evolution; Sun; White dwarf star
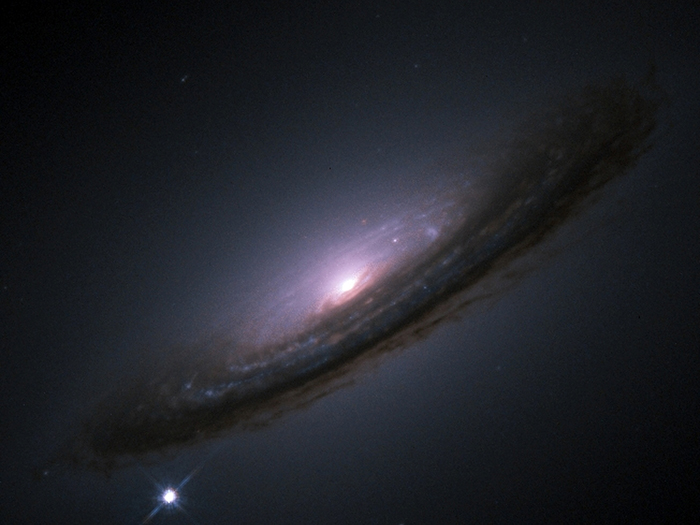
Candidate drivers for an accelerating universe
By combining such measurements of supernova distances with results from galaxy clustering and from fluctuations in the cosmic microwave background, consensus has emerged that the universe at present has about 70% of its energy density in dark energy, about 25% in another poorly understand substance known as dark matter, and only 5% in the form of "normal" matter made of neutrons, protons, and electrons. See also: Dark matter; Electron; Elementary particle; Matter (physics); Neutron; Proton; Standard model
The dark energy could be a form of the cosmological constant. German-born U.S. theoretical physicist Albert Einstein introduced this term into his theory of general relativity, still the prevailing theory of gravitation, shortly after he first proposed it in 1916. The cosmological constant balances the attractive force of gravity with a kind of repulsion to produce a static, stable universe. Hubble's later discovery of an expanding universe reportedly led Einstein to call the cosmological constant “the biggest blunder” of his life. Nevertheless, continued development into the concept of a cosmological constant, especially in light of quantum mechanics, now posits it as an energy density inherent to the vacuum of space, which creates a negative pressure that on cosmological scales could manifest as dark energy. See also: Cosmological constant; Gravitation; Quantum mechanics; Relativity
Einstein's cosmological constant is far from a perfect match with observations, however. Problematically, when the quantum principles that precisely characterize the fundamental force of electromagnetism in quantum electrodynamics are applied to the force of gravitation, the result is that the observed amount of dark energy is about 10120 times smaller than the predicted amount. This is a clue that some important theoretical aspect of gravitation is not well understood. One approach to addressing this problem is via inflationary cosmology, which proposes that the universe underwent an exponential expansion in the earliest moments after the big bang. If this is the case, it is possible that our particular inflated universe is just one of a potentially infinite number composing a “multiverse.” Different universe might exhibit different values for the cosmological constant, only some of which would lead to a universe like the one we inhabit. See also: Fundamental interactions; Inflationary universe cosmology
Another possibility is that general relativity is not precisely the correct theory of gravity. In that view, some aspects of cosmic acceleration could be attributed to the properties of modified gravity. These modified gravity theories also make predictions for the growth of structure in the universe; by carefully observing the growth of galaxy clustering, we can find out whether the data do or do not favor any deviation from Einstein's gravity.
Yet another possibility is that the dark energy is not something that is constant in time, as the cosmological constant would be, but something that has been changing its effects on expansion as the universe unfolds. This property could be detected by investigating the history of cosmic expansion with very precise observations of distant and nearby supernovae, as well as galaxy clusters. This observational campaign is ongoing and continues to improve in its scope and precision. Dark energy is still so new, so important, and so mysterious that it will be the focus of strenuous efforts in observational astronomy for decades to come. See also: The Dark Energy Survey and Camera