Key Concepts
The smallest unit of a compound, which consists of atoms bonded together in a unique arrangement. A diatomic molecule consists of two atoms linked together by chemical bonds. Examples include the oxygen and nitrogen of the air (O2 and N2, respectively) and the neurotransmitter nitric oxide (NO). A polyatomic molecule consists of more than two linked atoms. Examples include water (H2O), carbon dioxide (CO2; Fig. 1), ethanol (C2H5OH), and the vast molecule of deoxyribonucleic acid (DNA) with thousands of atoms.
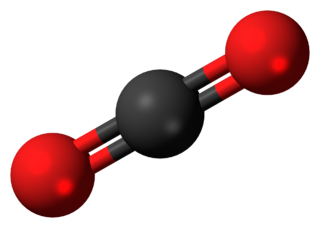
The bonds that hold the atoms together are covalent. That is, they consist of pairs of electrons that are shared by the neighboring atoms. This type of bonding is in contrast to ionic bonding, in which there is complete transfer of one or more electrons from one atom to another and large numbers of the resulting ions (charged atoms) clump together, as in sodium chloride (NaCl). Some chemists refer to the smallest unit of an ionic compound (in the case of sodium chloride, a sodium ion, Na+, and a chloride ion, Cl−) as constituting a “molecule” of the compound, but this usage is uncommon and the term “formula unit” is preferred. In this article, coverage is confined to covalently bonded molecules, of which there are millions of kinds. See also: Chemical bonding; Ionic crystals
Organic and inorganic molecules
Many molecules are organic. That is, they contain at least one atom and commonly many atoms of carbon. Molecules that do not contain carbon, with a few exceptions, are inorganic. Thus ethanol consists of organic molecules, and water consists of inorganic molecules. The principal exception to this general classification is carbon dioxide, which is treated as an honorary inorganic compound. The term “organic” arose when it was thought erroneously that such compounds could be produced only by living organisms. Advances in chemistry over decades have blurred the distinction between organic and inorganic, and many compounds are now known which, though they contain carbon, are regarded as lying within the domain traditionally regarded as inorganic chemistry (the so-called organometallic compounds), and many organic compounds that are actually produced by organisms contain metal atoms (some enzymes and vitamins, such as vitamin B12, which contains cobalt atoms). Nevertheless, the distinction between organic and inorganic is valuable as a broad-brush classification and is still widely used. This article concentrates on organic molecules. See also: Organometallic compound; Vitamin B12
Multiplicity of bonds
The bonds between atoms may be single, double, or triple (in rare cases, quadruple), in which neighboring atoms share one, two, or three electron pairs. The OH bonds in H2O are single (represented as HO
H), the CO bonds in CO2 are double (represented as O
C
O), and the NN bonds in N2 are triple (N
N). Broadly speaking, the greater the multiplicity of bonds, the more tightly bonded the atoms. This tightness of bonding is manifest, for example, in the inertness of molecular nitrogen and its role as a diluent of the dangerously reactive molecular oxygen of the atmosphere. It also accounts for the difficulty of converting atmospheric nitrogen into ammonia and nitrate fertilizers for use in agriculture.
Shapes
Molecules have not only a characteristic atomic composition but also, because the atoms are linked together in a characteristic array, a characteristic shape. Thus, H2O is an angular molecule in which the two OH bonds make an angle of about 104° to each other, and CO2 is linear (all three atoms in a straight line). The four bonds that carbon commonly forms (as in methane, CH4) are typically arranged in a tetrahedral array with bond angle close to 109°. The resulting shape of the ethanol molecule is seen in Fig. 2, which shows both the bonds as sticks joining balls representing the atoms (Fig. 2a), and a “space-filling” molecule (Fig. 2b), which gives a better notion of the overall bulk of the molecule by representing each atom by a sphere that corresponds to its actual size. Computer graphics are enormously useful for displaying molecular shapes, and can take a variety of forms. Figure 2c aims to display the general shape of the molecule without distinguishing the individual atoms.
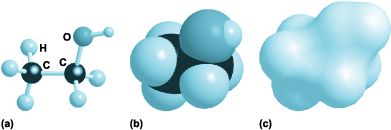
Flexibility and rigidity
The tetrahedral geometry of the bonds to carbon applies only when all the bonds are single. Moreover, neighboring groups of atoms are free to rotate around a single bond, which acts as a miniature axle. As a result, a polyatomic molecule consisting of many singly bonded atoms should not be thought of as a rigid framework, but as ceaselessly writhing and twisting into different shapes or “conformations.” Figure 3 shows two of the conformations of the hydrocarbon molecule octane (C8H18), a component of gasoline. See also: Conformational analysis
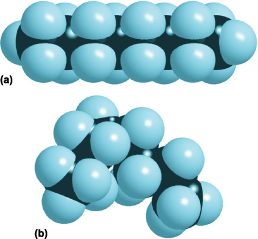
In contrast, the presence of a multiple bond confers structural rigidity because the neighboring groups of atoms joined by a double bond are not free to rotate relative to one another. This structural rigidity has a number of important consequences. For instance, beef fat consists of molecules (tristearin) in which three 18-carbon-atom-long chains are joined together. As all the bonds are single, these long chains are flexible and the entire molecule can roll up into a ball; these ball-like molecules can pack together closely, and tristearin is a solid. On the other hand, similar molecules produced in olives have a double bond in each of their chains. As a result, the chains are less flexible, the molecule cannot roll up into a ball, the molecules cannot pack together as closely, and the compound is an oil rather than a fat. See also: Fat and oil
Role of double bonds between carbon atoms
Double bonds between carbon atoms play important roles in physiology and industry. The primary act of vision, for instance, can be traced to the properties of double bonds. The retinas of human eyes are populated by the molecule cis-retinal (Fig. 4a). As can be seen from the line structure, one feature of the molecule is the alternation of single and double bonds between neighboring carbon atoms. This alternation has two consequences: the pairs of electrons forming the bonds are quite loosely attracted to the atomic nuclei and can easily be moved around by incident light; and the molecule is rigid and adopts the shape shown in Fig. 4b. However, when light is incident on the molecule, one of the double bonds is weakened, the long side-chain becomes free to rotate, and the molecule snaps into the form known as trans-retinal (Fig. 4b). This molecule can no longer fit into the protein molecule that normally houses it, and it is expelled. The protein molecule responds by relaxing into a new shape, and as a result a signal is pulsed along the optic nerve into the brain, which interprets it as “vision.” Various biochemical reactions then take place to restore the molecule to its original shape, in which it is primed to act again. See also: Photoreception
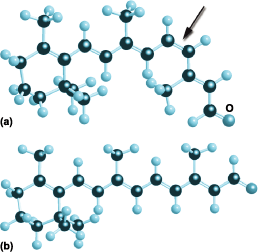
Polymerization
The industrial importance of double bonds between carbon atoms lies in the reactivity they confer on organic molecules (in contrast to the inertness conferred on nitrogen). One aspect of this reactivity is the ability to combine small double-bonded organic molecules into long chains. That is, certain molecules act as monomers that may be polymerized to form the plastics characteristic of the modern world. The process is illustrated in Fig. 5, which shows a variety of polymers based on ethene (ethylene, CH2 CH2). The most primitive of these polymers, but far from being useless, is polyethylene itself, the polymer of ethylene, which consists of long chains of thousands of
CH2
CH2
groups, often with branch lines where the polymerization has proceeded in a slightly different way. When the atom marked X in Fig. 5a is replaced by a chlorine atom to give the monomer CHCl
CH2, the polymerization process results in chains of
CHCl
CH2
groups and the polymer poly(vinyl chloride) [PVC]. When X is a benzene-like ring, the monomer is styrene and the resulting polymer is polystyrene. Chemists are able to modify the monomers in a wide variety of ways and to produce polymers with a correspondingly wide range of properties. Thus, if all the hydrogen atoms of the monomer are replaced by fluorine atoms, to give the monomer CF2
CF2, the resulting polymer is polytetrafluorethylene (PTFE), used in nonstick coatings. See also: Ethylene; Polyfluoroolefin resins; Polymer; Polymerization; Polyolefin resins; Polystyrene resin; Polyvinyl resins; Styrene
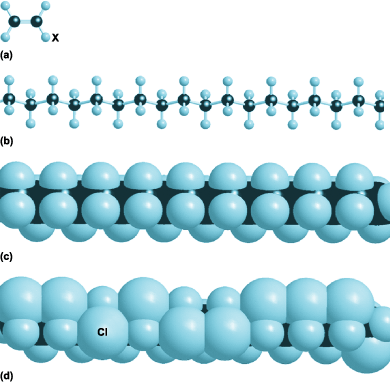
Aromatic compounds
The members of one very important class of molecules with double bonds have a greatly reduced reactivity (and thus are like organic counterparts of nitrogen). The parent of these so-called aromatic compounds is benzene, C6H6. Like benzene itself, many of these compounds have an aroma, but many do not, and the term applies to any compound that contains a benzene-like group. A benzene molecule consists of a planar hexagonal array of carbon and hydrogen atoms (Fig. 6). Although the carbon atoms are joined by alternating single and double bonds, and so might be expected to be highly reactive, this arrangement actually confers stability (for quantum-mechanical reasons) and the molecule is not very reactive. As a result, the ring is a motif that is found in many organic compounds, including polystyrene. See also: Aromatic hydrocarbon; Benzene
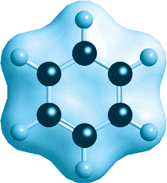
This brief survey only skims the subject of molecules. Whatever we breathe, eat, drink, and wear, whatever medications we take, whatever cosmetics we use, almost whatever we touch and see, is made of molecules.