On October 16, 2017, researchers announced for the first time the detection of gravitational waves and light coming from the same cosmic event. That event was the merging of two neutron stars, located about 130 million light-years from Earth. The findings usher in a new kind of “multi-messenger” astronomy, where combining traditional light observations with cutting-edge gravitational wave sensing will allow for a far deeper understanding of astrophysical phenomena. See also: Gravitational radiation; Observation of gravitational waves; Neutron star
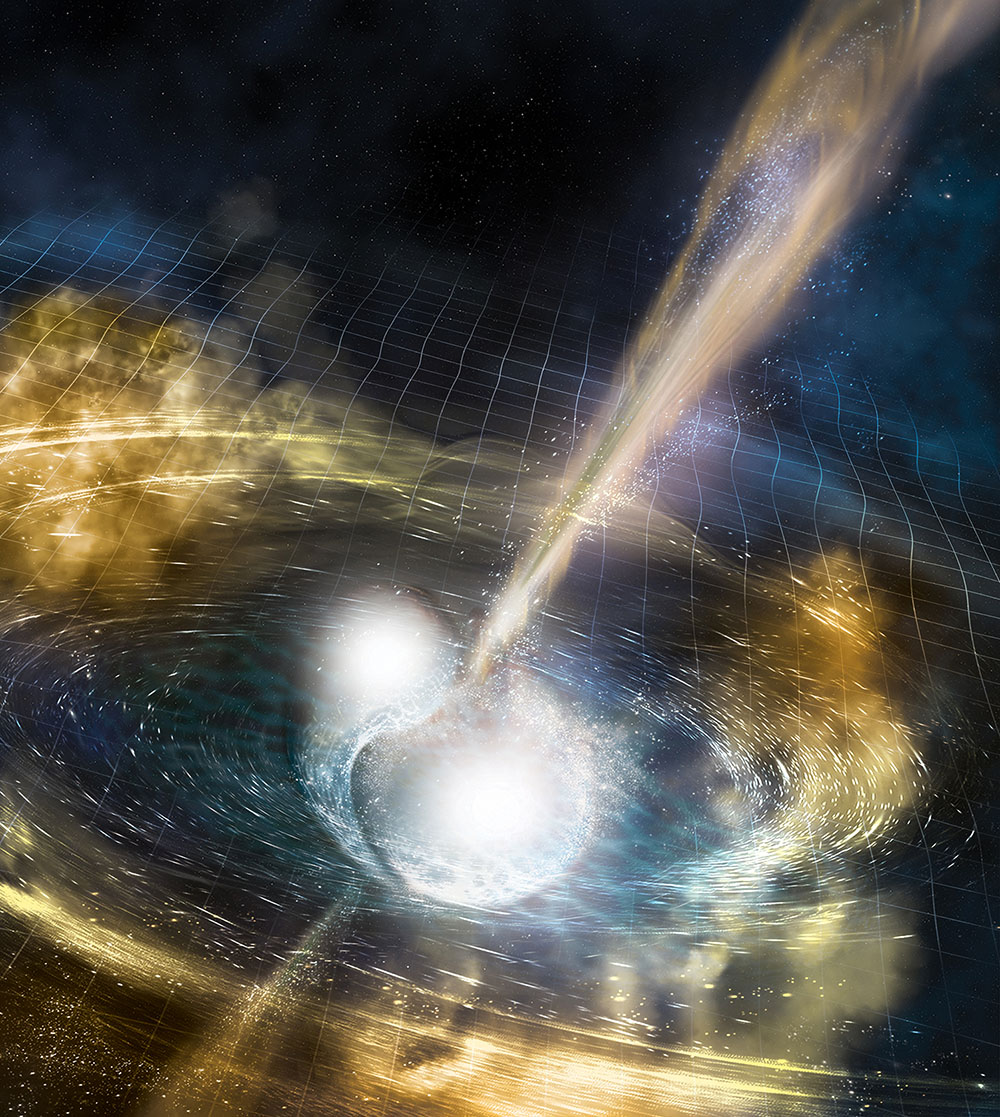
Gravitational waves are ripples in the fabric of spacetime generated by the spinning of massive objects. General relativity predicted the existence of the waves in 1916, but the instrumental sensitivity and precision required to detect these infinitesimal wrinkles in the universe did not become available until the LIGO (Laser Interferometer Gravitational-wave Observatory) experiment. Within LIGO, a laser is split and beamed down two 4-km- (2.5-mi-) long arms, oriented at right angles to each other. As gravitational waves pass through Earth, they compress and stretch the arms, throwing off the lasers’ precise alignment to astonishingly small, yet measurable levels—on the order of ten-thousandths the width of an atomic nucleus. LIGO made the first direct detection of gravitational waves in September 2015; its principal scientists were awarded the 2017 Nobel Prize in Physics. See also: Gravitation; LIGO (Laser Interferometer Gravitational-wave Observatory); Nobel Prizes for 2017; Relativity; Spacetime
The three LIGO detections to date all matched theoretical predictions for black holes colliding billions of light-years away, but with no detectable emissions of light to further study and verify the events. That changed on August 17, 2017. At 8:41 A.M. Eastern Daylight Time, LIGO’s twin detectors in Louisiana and Washington state, which serve as cross-checks on each other, registered a different type of gravitational wave signal, lasting 100 seconds instead of a handful of seconds as with black holes. A newly inaugurated gravitational wave detector in Italy, called VIRGO, also captured the signal. The three detections allowed scientists to triangulate the position on the sky where the waves had come from, in the southern constellation of Hydra. See also: Black hole; Constellation
An alert went out to ground- and space-based telescopes to scan this celestial region for any new sources of light in the instruments’ respective wavelength ranges. Data were recorded at around 70 observatories and, when pieced together with the gravitational wave signals, paint a remarkably detailed picture of the cataclysmic, energetic collision of two neutron stars. These objects, the approximately city-sized remnants of massive stars that exploded as supernovae, are incredibly dense; a single teaspoonful of their contents would have a mass of a billion tons. See also: Stellar evolution; Supernova
The observations show that the pair of neutron stars spiraled in toward one another under the force of gravity, speeding up and sending out gravitational waves. Upon their merging, a bright flash of intense gamma rays was unleashed, recorded by the Fermi Gamma-ray Space Telescope as a so-called short gamma ray burst. Until now, the origins of these powerful flashes had been unclear. After the neutron stars collided, a kilonova explosion occurred, scattering their contents into space and forging elements including gold and platinum, whose luminous signatures were picked up through further observations. Astronomers have struggled for decades to explain the cosmic origin of around half of the elements heavier than hydrogen, but now this case looks closed as well. See also: Fermi Gamma-ray Space Telescope; Gamma-ray bursts; Nucleosynthesis