Key Concepts
The biological, physical, and chemical processes involved in the cycling of phosphorus in the environment. The cycle comprises a geological (long-term) cycle and a biological (short-term) cycle, the latter with both terrestrial and aquatic components (Fig. 1). The phosphorus cycle differs markedly from other nutrient cycles (carbon, nitrogen, oxygen, and hydrogen), because phosphorus has no significant gaseous phase and, therefore, no major atmospheric component to its cycle. See also: Biogeochemistry; Phosphorus
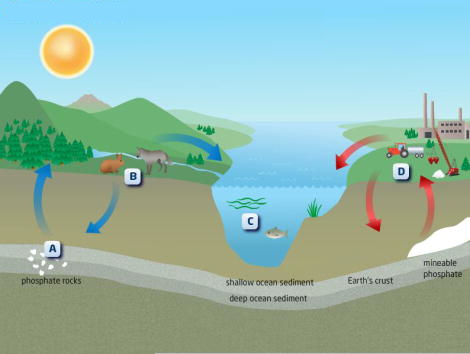
Phosphorus is indispensable to life on Earth, as it is involved in the passage of genetic information, energy transfer, and the construction of plant cells. Despite this, the amount of phosphorus available for biological uptake is relatively small, so productivity in many terrestrial and aquatic ecosystems is often limited by phosphorus availability. As a result, human interference in the phosphorus cycle can have severe environmental consequences. For example, phosphorus pollution of water bodies by sewage effluents and drainage from agricultural land can contribute to the growth of toxic blue-green algae, fish deaths, and a drastic reduction in the quality of affected water bodies. See also: Plant cell
Geologic cycle
In the natural environment, phosphorus usually exists as fully oxidized phosphate. While the phosphorus cycle has only a negligible atmospheric component, the input of phosphorus from the atmosphere can be significant in some nutrient-poor (oligotrophic) ecosystems.
The generalized geological phosphorus cycle is shown in Fig. 2. It begins with the slow weathering and dissolution of phosphate minerals (mainly apatite) in the terrestrial environment. In the short term the released phosphorus is involved in the soil-plant-animal system (the terrestrial biological cycle), but in the long term it leaches slowly from the soil and is transported by rivers to the oceans. There it can be taken up by phytoplankton and then enters the aquatic biological cycle, or it can precipitate with calcium to form insoluble calcium phosphates, which are deposited onto the ocean floor. The sediments are converted to sedimentary rock by burial and diagenesis (chemical and physical changes) over millions of years, and subsequently uplifted, whereby the process begins again. See also: Apatite; Diagenesis; Phosphate minerals
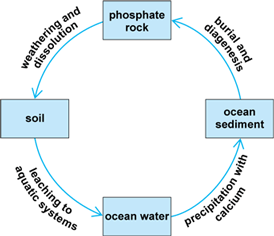
An alternative path in the cycle results in the deposition of phosphorus as guano following movement through the marine food chain (phytoplankton–fish–birds). Notable guano deposits exist on Pacific coastal regions and islands, although these are being rapidly depleted by mining. See also: Marine ecology
Biological cycle
The slow release of phosphorus from mineral sources means that the biological demand for phosphorus far exceeds the amounts available for biological uptake. As a result, the availability of soil phosphorus plays a central role in determining the ecology of terrestrial ecosystems. See also: Soil chemistry; Terrestrial ecosystem
The biological phosphorus cycle in the terrestrial environment is shown in Fig. 3. Plants take up phosphorus from the soil solution as inorganic orthophosphate, but the availability of this compound in soil is typically low. In the early stages of pedogenesis (soil formation), phosphorus is released slowly by the weathering and dissolution of apatite. However, inorganic phosphorus reacts strongly in the soil, forming precipitates with calcium, iron, and aluminium, and becoming adsorbed on the reactive surfaces of clay minerals. This means that the amount of soluble phosphorus in soil solution is typically only a minute fraction of that present in the whole soil. In a kilogram of soil, for example, no more than 0.0001 g of phosphorus will typically be dissolved in the soil solution, whereas approximately 1 g will be contained in the solid soil. This does not mean that plants cannot access phosphorus from the soil, because as they deplete inorganic orthophosphate from the soil solution, it is replenished from the solid phase, thus maintaining relatively constant, albeit low concentrations of phosphorus available to plants. The extent to which solid-phase phosphorus is available to plants can be determined by extraction using a variety of chemicals, including water, sodium bicarbonate (Olsen's reagent), and various acids. See also: Weathering processes
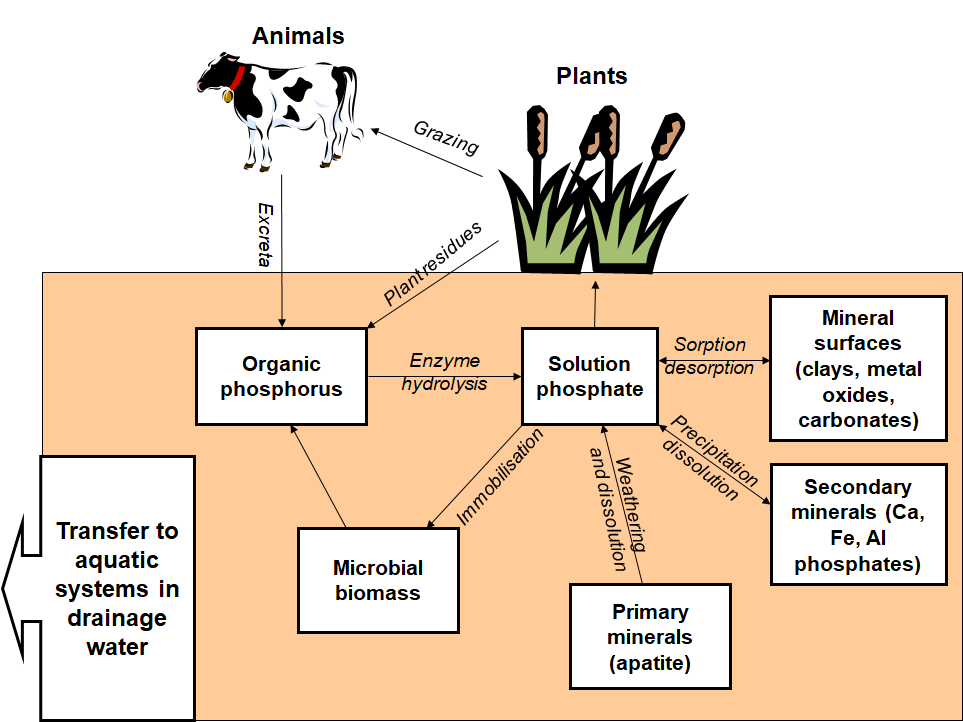
After uptake from the soil, plants convert inorganic orthophosphate to organic forms of phosphorus (phosphorus in combination with carbon structures), such as nucleic acids and phospholipids. Therefore, phosphorus is returned to the soil in plant residues as organic phosphates. These organic compounds often represent the major soil phosphorus fraction in natural systems, so it is not surprising to find that many organisms possess complex mechanisms for accessing phosphorus from them. In particular, they are hydrolyzed by enzymes called phosphatases, released by plants and microorganisms in response to a deficiency in phosphorus. The conversion of organic phosphorus to inorganic forms (mineralization) is, therefore, a key stage in the biological phosphorus cycle. See also: Nucleic acid; Phosphatase; Phospholipid
Phosphorus availability also frequently limits biological productivity in natural fresh-water systems. The situation is somewhat different in estuarine environments, which tend to be limited more by nitrogen availability, while marine systems can be either phosphorus- or nitrogen-limited. In the oceans, there can be significant differences in phosphorus concentrations with depth; biologically productive surface layers generally contain smaller phosphorus concentrations than the deeper waters.
Phosphorus in plant tissues
Prokaryotic and eukaryotic cells require a certain concentration of phosphorus for normal function. This cellular phosphorus is required for the synthesis of deoxyribonucleic acid (DNA), ribonucleic acid (RNA), proteins, carbohydrates, lipids, and energy transfer. However, a clear distinction must be made between cellular and stored phosphorus when considering the role of plants in the phosphorus cycle. Plant leaf tissues, which typically have no reproductive function, contain between 1 and 10 mg of cellular phosphorus per gram of dry tissue. Since basic structure and function is essentially similar for all cells, concentrations of cellular phosphorus under normal circumstances typically do not vary more than an order of magnitude. Cellular phosphorus in plants varies in response to the biological availability of soil phosphorus and to the genotype of the plant. Genetic factors include variations in the plant's genetic background (genome), and allelic variation in specific genes that affect phosphorus uptake and distribution throughout the plant. Long-lived plants such as perennials are often adapted to survive on soils containing small concentrations of biologically available phosphorus, and have evolved strategies to retain phosphorus following uptake, called retentive strategies. In contrast, short-lived plants such as most annual grain and legume crops are adapted to soils with greater concentrations of available phosphorus and have evolved (or been selected for) an acquisitive strategy. Stored phosphorus can exceed the concentration of cellular phosphorus by several times during certain stages in plant growth. Prokaryotic cells (such as bacteria) synthesize linear-chain polyphosphates, whereas eukaryotic cells (such as those in developing plant seeds) synthesize and store phosphorus as a compound called phytic acid (Fig. 4). Synthesis and breakdown of stored phosphorus may also maintain relatively constant cellular phosphorus concentrations, a process called phosphorus homeostasis. Whereas nonreproductive tissues may accumulate between 1 and 5 mg of phosphorus per gram of tissue, reproductive tissues, such as mature seeds, may accumulate between 5 and 20 mg. See also: Deoxyribonucleic acid (DNA); Eukaryota; Plant tissue systems; Phosphate metabolism; Prokaryote; Ribonucleic acid (RNA)
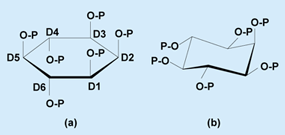
Strategies of plant phosphorus acquisition
Terrestrial plants acquire phosphorus from the soil through their roots, which are adapted to the complexities of phosphorus availability in soils. Roots take up mineral nutrients directly but also access them via symbiotic partnerships with bacteria or fungi. In the case of phosphorus, roots can take up only soluble inorganic orthophosphate, but frequently form symbiotic relationships with mycorrhizal fungi, which grow into the roots from the soil, thus increasing the total volume of soil from which plants can obtain phosphorus. The fungus receives a labile carbon substrate in return. While a small number of plant species form bacterial symbioses important for nitrogen fixation, the majority of terrestrial plants form symbioses with mycorrhizal fungi that increase their uptake of phosphorus, reflecting the importance of phosphorus in most terrestrial environments. Other strategies that increase phosphorus uptake from the soil include the secretion of enzymes that liberate phosphorus from organic compounds, the extrusion of protons and organic acids that acidify the soil and solubilize both inorganic and organic phosphorus, and the alteration of the kinetics of root plasma-membrane phosphorus transporters to enhance the ability of roots to take up phosphorus. However, these strategies are often employed by specialist species, such as those that invade disturbed sites, whereas mycorrhizal symbiosis is common to plant species inhabiting mature soils throughout the world. See also: Mycorrhizae; Soil; Soil ecology; Soil fertility; Rhizosphere; Rhizosphere ecology
Phosphorus storage in plants
The limited availability of phosphorus in the environment means that plants have evolved strategies to conserve phosphorus once it has been acquired from the soil. These include the ability to translocate phosphorus from older tissues to younger or reproductive tissues. Most agricultural production involves the cultivation of seed crops such as cereal grains and legumes. A significant fraction of the phosphorus taken up by these annual plants is ultimately stored in seeds, of which about 75 percent occurs as phytic acid. This single compound, therefore, represents a major bottleneck in the flux of phosphorus in the agro-ecological phosphorus cycle. Indeed, phytic acid in seeds represents a sum equivalent to more than half of the total amount of phosphorus fertilizer applied annually in world agriculture. Phytic acid is only a small fraction of the total phosphorus returned to the soil in plant residues, but it reacts strongly in most soils and accumulates relative to other forms of soil organic phosphorus. As a result, phytic acid is the single most abundant soil organic phosphorus compound and can also be important in some aquatic systems.
Human impact on global cycle
As with almost all natural element cycles, humans have interfered significantly in the global phosphorus cycle (Fig. 5). Phosphorus is mined from phosphate rock and guano deposits (although the latter are rapidly being depleted) and is used for a wide range of purposes, including the manufacture of detergents, fertilizers, and food additives.
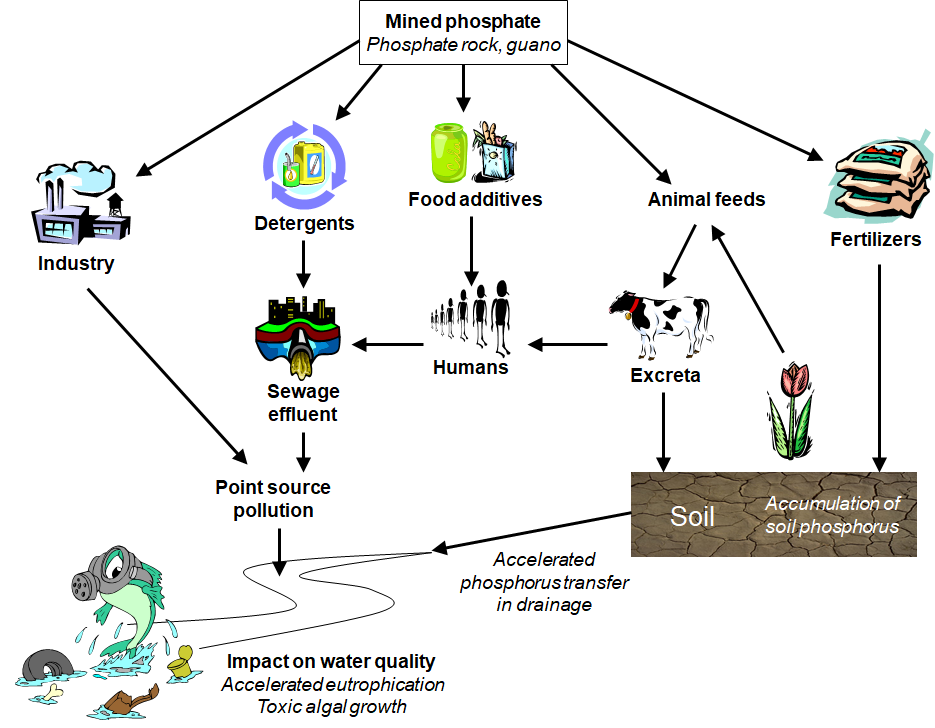
In agricultural systems, phosphorus is removed from the soil in crops and animal products, so must be replaced to avoid phosphorus deficiency. Phosphorus deficiency is widespread on a global scale, notably in Australia, South Africa, and parts of South America. To maintain soil phosphorus at levels required to maintain crop yields, mineral phosphate fertilizer is applied to the land, along with other essential plant nutrients including nitrogen and potassium. Only a small proportion of the applied phosphorus fertilizer becomes available for crop uptake in any one year, so phosphorus must be continually applied to maintain yields. In the long term, however, phosphorus accumulates in the soil to levels that exceed those required by the crop. This is likely the case where animal operations import additional phosphorus into the system via feed products. See also: Fertilizer
The accumulation of phosphorus in soil has severe environmental implications, because it accelerates the rate at which phosphorus leaks into aquatic systems through drainage water. The transfer of soil phosphorus in land drainage is rarely significant relative to the large quantities of phosphorus in the soil, and was considered agronomically unimportant for many years. However, even small amounts of phosphorus can dramatically alter the trophic status of water bodies, because the availability of phosphorus frequently limits the biological productivity of such systems. Phosphorus in effluents from sewage treatment works and industrial sources (point sources) also contributes to phosphorus pollution of aquatic systems, but such sources are relatively easily isolated and abated. For example, modern sewage treatment plants in sensitive catchments now involves tertiary treatment to remove nutrients from the effluent. The result is that phosphorus transfer in drainage from agricultural land (diffuse pollution) is currently the major source of phosphorus pollution to aquatic environments. See also: Sewage treatment
Phosphorus pollution of aquatic systems can contribute to serious health problems for both animals and humans, and can be extremely costly in financial terms. While phosphorus is not directly toxic, phosphorus pollution can accelerate the process of eutrophication in receiving water bodies. This process is associated with such undesirable consequences as the growth of toxic blue-green algae (cyanobacteria), oxygen depletion, and fish deaths. A phosphorus-polluted water body is often foul-smelling, the water is expensive to treat for potable supply, and its quality is severely reduced. Concerns over human health include potential neurological damage from the dinoflagellate Pfiesteria piscidia, notably in the Chesapeake Bay area of the eastern United States. Diffuse nutrient pollution and associated eutrophication are among the greatest threats to global water quality, and many developed countries now suffer acute effects of eutrophication in major water bodies. Notable examples of eutrophication linked to diffuse phosphorus pollution have been documented in the United States (Chesapeake Bay and Lake Okeechobee), Australia (Gippsland Lakes and Murray-Darling river system), as well as many parts of Europe, such as the Netherlands, where intensive livestock operations have caused extreme accumulation of phosphorus in the soil. See also: Cyanobacteria; Dinoflagellida; Eutrophication
Role of phytic acid
There is much interest in seed phytic acid (Fig. 4) from an agronomic perspective, in terms of both human and environmental health. Humans and nonruminant farmed animals (poultry, swine, and fish) do not efficiently digest and absorb phosphorus from phytic acid present in grain- and legume-based foods and feeds. Dietary phytic acid forms strong complexes with minerals such as calcium, iron, and zinc while passing through the digestive tract, and also complexes with proteins. These salts, which are largely excreted, have potentially negative outcomes in both human nutrition and animal production. In terms of human nutrition, phytic acid can contribute to iron and zinc depletion and deficiency, especially in populations that depend on cereal crops and legumes as staple foods. To put this in context, iron deficiency anemia is among the most significant public health problems throughout the world. Reducing the intake of dietary phytic acid could help alleviate iron and zinc deficiency.
In the context of livestock production, the excretion of feed phytic acid by nonruminants means that >50 percent of the total phosphorus in the feed is not utilized but ultimately ends up in animal manures. Since phosphorus is an important nutrient, feeds must be supplemented with a more readily available form. The phytic acid that ultimately ends up in the feces represents a significant environmental problem, because the high phosphorus concentrations can contribute to eutrophication if it escapes from the soil in runoff water. To put this aspect of the phytic acid problem in perspective, about 80 percent of all maize (corn) grown in the United States is used in livestock feeds, and about 50 percent of that is used in poultry and swine feeds. Since phytic acid constitutes about 75 percent of the phosphorus in maize grain, around 30% of all phosphorus in maize grain is excreted and can potentially contribute to water pollution. See also: Water pollution
Remediation of phosphorus pollution
Remediation of the effects of phosphorus pollution is a complex problem. Point sources of phosphorus, including sewage and industrial effluents, can be effectively isolated and abated, but diffuse pollution is much more difficult to control. Remedial efforts are often thwarted by processes occurring within the affected water body, because phosphorus remaining in lake sediments can sustain elevated concentrations of phosphorus in the water column for many years following reductions in phosphorus pollution from the surrounding catchment.
A large research effort has been devoted to understanding the process of phosphorus transfer from the soil system. However, the complexity of phosphorus movement in the landscape means that there is still no definitive understanding of the process. Problems include differences in the relative biological availability of the variety of chemical and physical forms of phosphorus, transformations that occur during transport, and difficulties involved with scaling-up information on processes active in small-scale studies to those controlling phosphorus dynamics in the watershed.
Sustainable farming systems involve minimal or no use of mineral fertilizers by emphasizing the use of organic manures and the recycling of nutrients. Such systems are attractive from an environmental standpoint because they minimize the accumulation of soil phosphorus that can contribute to the pollution of aquatic systems. However, such practices can be difficult to implement on a large scale in an economically viable way. See also: Agricultural soil and crop practices
An approach to remediate phosphorus pollution from agricultural land in the United States is the Phosphorus Index. This simple procedure involves the determination of an index value for individual fields based on the risk of phosphorus transfer to a stream or river. The index values are based on parameters such as phosphorus concentration in the soil, landscape factors related to the likelihood of water movement to streams, and the rate of fertilizer and manure application. Remedial action, such as reducing fertilizer application, conservation tillage practices, or construction of buffer strips, can then be taken for fields most at risk of contributing to phosphorus pollution.
Animal manures have been implicated as a major source of diffuse phosphorus pollution, illustrated by the fact that several European nations, the United States, and Canadian provinces now require that livestock producers deal with phosphorus in animal manures, including regulations that require a 40 percent reduction in waste phosphorus. Two main approaches are used to achieve these reductions. Phytase enzymes are currently produced industrially as a feed supplement to degrade phytic acid in the gut. This can increase phosphorus uptake by the animal by up to 50 percent with minimal side effects. An alternative strategy uses plant genetics and breeding to develop crops that produce low concentrations of phytic acid, called low-phytate or low-phytic-acid crops. Single-gene, low-phytic-acid mutations of maize, barley, wheat, rice, and soybean have been isolated, which contain from 50 to 90 percent less phytic acid in their seeds than the original varieties. Total phosphorus in the seed remains unaffected, so phosphorus that nonruminants can absorb and utilize is increased from approximately 25 percent to more than 75 percent of seed phosphorus (Fig. 6). Phosphorus excretion from animals fed low-phytic-acid grains is reduced by about 50 percent, which can be further reduced by combining the low-phytic-acid grains and legumes with phytase supplements.
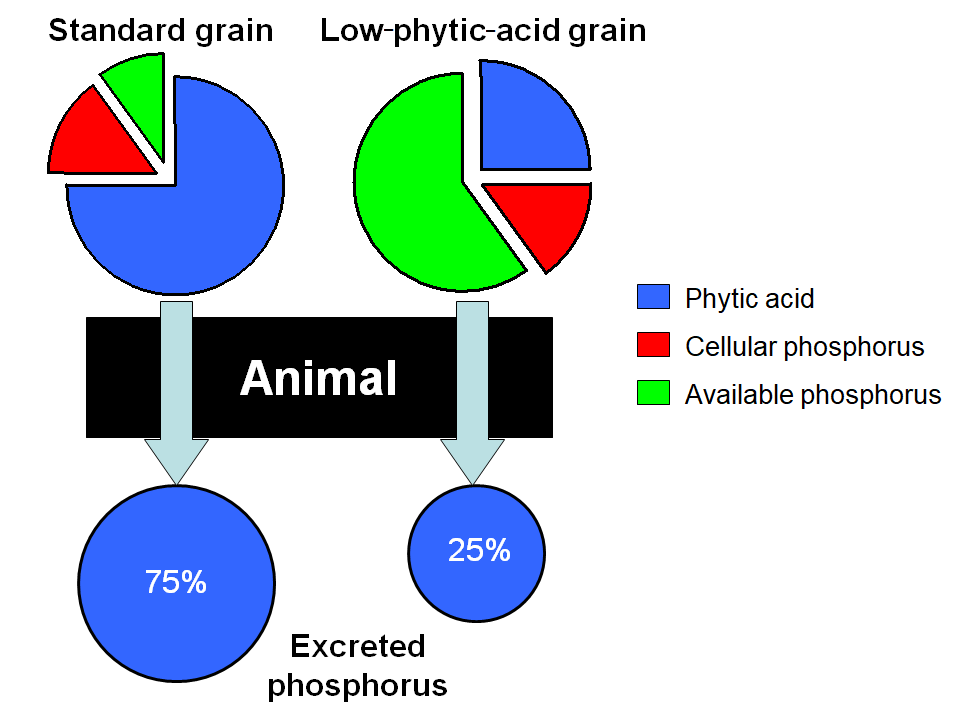