SUMOs (small ubiquitin-like modifiers) are ubiquitin-like proteins (Ubls) that become conjugated to substrates through a pathway that is biochemically similar to ubiquitination. Ubiquitination is the conjugation of ubiquitin (a 76-amino-acid protein) to cellular proteins and regulates a broad range of eukaryotic cell functions; in particular, it targets proteins for degradation. SUMOylation is involved in many cellular processes, including deoxyribonucleic acid (DNA) metabolism, gene expression, and cell cycle progression. Clinical studies suggest that SUMOylation plays important roles in disease processes (for example, diabetes, viral infection, and carcinogenesis). In this article, a number of topics will be discussed, including the basic mechanism of SUMO modification, the enzymes involved in the process, and how SUMOylation regulates substrate proteins.
SUMO paralogues and isoforms
Yeasts have only one SUMO protein (Smt3p in Saccharomyces cerevisiae and Pmt3p in Schizosaccharomyces pombe), whereas mammalian cells express four SUMO paralogues (SUMO1–4). Paralogues are genes that diverged through gene duplication, and it is anticipated that the isoforms encoded by these genes differ from each other in function. Among the mammalian SUMO proteins, SUMO1 has approximately 45% identity compared with SUMO2 or SUMO3, while the latter two are approximately 95% identical to each other. Because of this high degree of identity, they are collectively called SUMO2/3. SUMO1–3 proteins are expressed as precursors, with short peptides on their C-termini that must be removed to reveal a di-glycine motif that is required for conjugation (step a in the illustration). Although SUMO4 has a high degree of sequence identity compared with SUMO2 (approximately 86%), it is only expressed in a subset of tissues. SUMO4 is processed very inefficiently, and it is not clear whether it becomes conjugated to other proteins under physiological conditions; thus, the biological role of SUMO4 remains unclear.
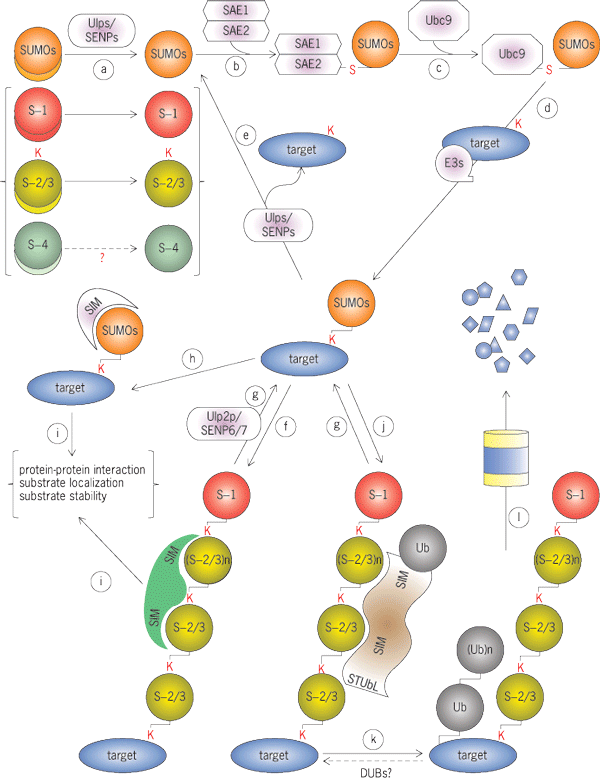
Like ubiquitin, SUMOs can form polymeric chains through the sequential conjugation of SUMOs to each other. In S. cerevisiae, the N-terminal domain of Smt3p contains three lysines (K11, K15, and K19) that may serve as internal conjugation sites. In vertebrates, lysine 11 (K11) of SUMO2/3 is primarily used during polymeric SUMO (poly-SUMO) chain extension. SUMO1 lacks a conserved lysine at the equivalent residue and might only become conjugated to the end of SUMO2/3 chains as a “chain terminator.”
SUMO conjugation pathway
SUMOylation is mediated through an enzyme cascade similar to ubiquitination (see illustration). Enzymes of this pathway are listed in the table. Mature SUMO is activated by the formation of a thiolester bond with SUMO activating enzyme (E1 enzyme) in an adenosine triphosphate (ATP)–dependent manner (step b). The SUMO E1 enzyme is a heterodimer composed of SAE1 and SAE2 (also known as Aos1 and Uba2, respectively). SUMO is transferred from SAE1/SAE2 to form a thiolester linkage with the unique SUMO conjugating enzyme (E2 enzyme), known as Ubc9 (step c). Ubc9 catalyzes the formation of an isopeptide bond between the SUMO protein and the substrate (step d). Isopeptide bonds formed in this reaction are chemically similar to peptide linkages assembled during protein translation, except that they link SUMO's C-terminal carboxyl group and the ε-amino of a substrate lysine side chain (rather than linking to an amino group of a previous amino acid within a polypeptide). There is some preference for lysine residues that reside within a consensus sequence, ψKXE, where ψ is a hydrophobic residue and X is any amino acid. However, many instances have been found in which lysines outside of such canonical sequences are utilized.
Activity |
Homo sapiens |
Saccharomyces cerevisiae |
Schizosaccharomyces pombe |
---|---|---|---|
SUMO isoforms |
SUMO1 |
Smt3p |
Pmt3p |
SUMO2 |
|||
SUMO3 |
|||
SUMO4 |
|||
Activating enzyme (E1) |
Uba2/SAE2 + Aos1/SAE1 |
Uba2p + Aos1p |
Uba2/Fub2 + Rad31 |
Conjugating enzyme (E2) |
Ubc9 |
Ubc9 |
Hus5 |
SP-RING SUMO ligases |
PIAS1 |
Siz1p |
Pli1 |
(SUMO E3) |
PIAS3 |
Siz2p/Nfi1p |
|
PIASXa |
|||
PIASXb |
|||
PIASy |
|||
Mms21 |
Mms21p |
Nse2p | |
Zip3p |
|||
Non-SP-RING SUMO |
Topors |
||
ligases (SUMO E3) |
RanBP2 |
||
PC2 |
|||
CBX4 |
|||
HDAC4 |
|||
Krox20* |
|||
Cyclin B1 interacting protein-1 (CCNB1IP1) |
|||
Stanniocalcin-1 (STC1) |
|||
TRIM family proteins |
|||
SUMO-targeted ubiquitin |
RNF4 |
Hex3p/Slx5p + Slx8p |
Rfp1p + Slx8p |
ligases (STUbLs) |
Rfp2p + Slx8p | ||
Ris1p/Uls1p |
Rad60 | ||
SUMO proteases |
SENP1 |
Ulp1p |
Ulp1p |
SENP2 |
|||
SENP3 |
|||
SENP5 |
|||
SENP6 |
Ulp2p/Smt4p |
Ulp2p | |
SENP7 |
|||
Wss1p |
|||
DeSUMOylating isopeptidase 1 (DeSI-1) |
|||
DeSI-2 |
|||
USPL1 |
*Activity demonstrated for mouse homologue.
Although Ubc9 can be sufficient for in vitro conjugation, in vivo conjugation typically involves SUMO ligases (E3 enzymes). E3 enzymes are likely to play a key role in determination of the overall spectrum of SUMOylated species, especially because conjugation of all SUMOs and all substrates utilize the same E1 and E2 enzymes. E3 enzymes facilitate conjugation either by bringing the Ubc9–SUMO thiolester and substrate together or by stimulating the transfer of SUMO from Ubc9 to the substrate. The number of known SUMO E3 ligases remains much smaller than those of ubiquitin E3 ligases. For some of these enzymes, specificity has been demonstrated for substrate recognition or for particular SUMO isoforms.
SUMO E3 enzymes can be broadly categorized into two groups. The first group is composed of SUMO E3 enzymes possessing RING-fingerlike domains (Siz/PIAS-RING; SP-RING). These enzymes are proposed to function in a manner analogous to ubiquitin RING E3 ligases, mostly by juxtaposition of substrates to the Ubc9–SUMO thiolester. This class of SUMO ligases is found in both yeast and vertebrates. The second class consists of a diverse and growing set of vertebrate proteins that are simply categorized by the absence of any SP-RING domain. The putative SUMO ligase domains of these proteins lack common features, and their enzymatic mechanisms are generally not well studied. Indeed, there is no reason to anticipate that they will utilize shared mechanisms given their structural diversity.
SUMO proteases and SUMO-targeted ubiquitin ligases
The collective activity of SUMO proteases enables SUMOylation to be a highly dynamic modification. Both post-translational processing (step a) and the deconjugation of SUMOylated species (steps e and g) are mediated by a family of SUMO proteases known as ubiquitin-like protein-specific proteases in budding yeast (Ulp1p and Ulp2p) or as sentrin-specific proteases in mammals (SENP1–3 and SENP5–7). By comparing their protein sequences, these SUMO proteases can be divided into two branches. Vertebrate SENP1, SENP2, SENP3, and SENP5 are most closely related to Ulp1p, whereas SENP6 and SENP7 are more closely related to Ulp2p.
Ulps/SENPs show distinct substrate specificities, and their activities are regulated by localization. Ulp1p and SENP2 are primarily localized to nuclear pores; in contrast, a number of patterns of SENP1 localization have been reported. Ulp1, SENP1, and SENP2 catalyze both deconjugation of SUMO and processing, whereas SENP1 and SENP2 act on SUMO1 and SUMO2/3. SENP3 and SENP5 concentrate within the nucleolus. In vitro, they are active in both processing and deconjugation reactions, and they have higher activity towards SUMO2/3 substrates. Ulp2p, SENP6, and SENP7 localize within the nucleoplasm. They are particularly active in trimming of poly-SUMO chains (step g), and SENP6 and SENP7 show a strong preference for SUMO2/3-conjugated species. Non-Ulp/SENPs have recently been reported as novel SUMO proteases, including a metalloprotease that may edit SUMO chains in budding yeast (Wss1p), as well as two vertebrate cysteine proteases (DeSI-1 and DeSI-2).
Poly-SUMO chains can be recognized by SUMO-targeted ubiquitin ligases (STUbLs) [step k]. STUbLs mediate ubiquitination of the poly-SUMOylated species, leading to their degradation by proteasomes (large proteolytic complexes that degrade intracellular proteins; step l). As mentioned earlier, poly-SUMO chains are trimmed by Ulp2p-related proteases, which thus antagonize this process.
Effects of SUMOylation on substrates
A large number of potential SUMOylation substrates have been identified through proteomic studies. SUMOylation promotes a variety of fates for individual substrates, frequently reflecting the gain or loss of protein–protein interactions upon SUMOylation. Many cellular proteins possess short SUMO-interacting motifs (SIMs) that form low-affinity interactions with SUMOs. These SUMO–SIM contacts promote recognition of SUMOylated species, sometimes in an isoform-specific manner (step i). Moreover, numerous proteins that recognize poly-SUMOylated species, such as Ulp2-related proteases or STUbLs, have many SIMs repeated in tandem.
Changes in substrate fate after SUMOylation include alterations of localization, stability, or activity. For example, SUMO modification of the PML (promyelocytic leukemia gene product) protein is important for the organization of subnuclear foci called PML nuclear bodies (PML-NBs), as well as for the recruitment of other proteins into PML-NBs. Furthermore, poly-SUMOylation of PML in response to arsenic trioxide treatment leads to its STUbL-mediated degradation. The budding yeast proliferating cell nuclear antigen (PCNA) is another well-studied substrate that can be modified by either ubiquitin or SUMO. Ubiquitination of PCNA is involved in DNA damage responses, whereas SUMOylation of PCNA mediates interactions with DNA helicase Srs2p (an enzyme that unwinds the DNA double helix) and prevents recombination during DNA replication.
Alternatively, SUMOylation can act in antagonism to ubiquitination in some cases. For instance, IκBα, a regulator of the transcription factor NF-kB, can be SUMO modified on its lysine (K21). This modification either competes with ubiquitin conjugation on the same lysine or blocks the access of another ubiquitin conjugation site and therefore prevents IκBα degradation.
SUMO and diseases
SUMOylation has been implicated in human diseases. SUMOylation substrates play prominent roles in neurodegenerative diseases, including SOD1 in amyotrophic lateral sclerosis; huntingtin in Huntington's disease; ataxin-1 in spinocerebellar ataxia type 1; tau, α-synuclein, and DJ-1 in Parkinson's disease; and tau and APP in Alzheimer's disease. Additionally, Ubc9 polymorphisms may be associated with an elevated risk of Alzheimer's disease in Korean patients. Similarly, non-SUMOylatable mutants of lamin A (a type of structural protein) are associated with familial dilated cardiomyopathy and conduction system disease. A single mutation in SUMO4 (M55V) is associated with an increased risk of type-1 and type-2 diabetes. The reasons for this phenotype are not obvious, however, given that (1) SUMO4 messenger RNA (mRNA) is mainly expressed in kidneys and (2) its extent of conjugation may be limited.
Studies in many organisms indicate that SUMOylation is required for genomic stability, transcriptional regulation, and cell cycle control, all of which are important factors in carcinogenesis. Moreover, dysregulated SUMOylation has been observed in human cancers. For example, elevated Ubc9 levels have been found in ovarian, cervical, prostate, and lung cancers, as well as in multiple myelomas, whereas persistent elevations of SENP1 and SENP3 levels have been found in prostate cancers. These observations may indicate that SUMOylation and de-SUMOylation must be carefully balanced; thus, the SUMO pathway may be a potential target for cancer therapy. Consistent with this idea, arsenic compounds have been effectively used to treat acute promyelocytic leukemia (APL) patients in Chinese traditional medicine. The discovery of how PML is controlled by SUMOylation provides a molecular mechanism for understanding the effectiveness of this treatment. Recent reports also suggest that deregulation of poly-SUMO chain formation may promote sensitivity to genotoxic stress caused by radiation or chemotherapy in cancer cells.
Conclusions
The understanding of SUMO modification has greatly expanded since its discovery, and many basic principles through which it operates have been determined. Nevertheless, many aspects of SUMO regulation and function remain to be explored. The answers to these questions will greatly help in the comprehension of cell function as well as providing clinical targets for disease treatments.
See also: Biochemistry; Cancer (medicine); Cell biology; Chemotherapy and other antineoplastic drugs; Gene; Proteasome; Protein; Protein degradation; Protein engineering; Ubiquitination