A fundamental innovation that distinguishes eukaryotes from bacteria is the way in which the genome is organized. In bacteria, DNA is a naked circular molecule. In eukaryotes ranging from yeast to humans, DNA is packaged into chromatin, which is composed of beadlike structures called nucleosomes. Each nucleosome contains two copies of the histone proteins, H2A, H2B, H3, and H4, and wraps 147 base pairs (bp) of DNA in a left-handed ramp, resulting in a conserved octameric structure (Fig. 1). In addition to packaging DNA, nucleosomes can impede access to regulatory regions and genes. This is referred to as epigenetic control because it provides a layer of information above the genetic code, which itself is embedded within the DNA sequence. The epigenetic status of the genome plays a crucial role in regulating gene expression, DNA repair, DNA accessibility, and DNA segregation. Consequently, it has enormous significance in the biology of normal and diseased cells.
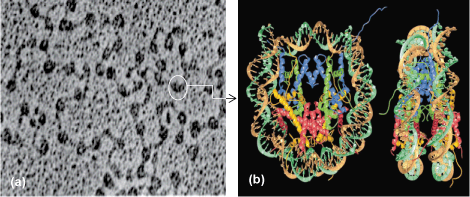
Histone variants, epigenetics, and mitosis
One key example of histones serving an epigenetic function is at cell division during mitosis. At mitosis, a single region on each chromosome is responsible for ensuring accurate segregation of the newly replicated DNA into two daughter cells. At this unique location called the centromere, a centromere-specific histone H3 variant called CenH3 replaces canonical histone H3 within nucleosomes. CenH3 nucleosomes provide the platform on which a large complex of proteins called the kinetochore assembles. The kinetochore, in turn, binds to spindle microtubules, drawing the newly replicated DNA molecules into the two daughter cells. To ensure that chromosomes do not break as a result of multiple microtubule attachments, it is imperative that CenH3 is strictly restricted to a single site per chromosome. Conversely, depleting CenH3 from the cell results in mitotic arrest and cell death, because the kinetochore complex fails to associate with the chromosome. Taken together with the observation that no unique DNA sequence appears necessary for centromere function, it is thought that the primary determinant for centromere specification is the CenH3 nucleosome itself. Therefore, centromeres are considered a prime example of epigenetic specification and inheritance. An example of the consequences of epigenetic dysfunction related to CenH3 is seen in human colorectal cancers. In these diseased cells, CenH3, rather than being restricted to one site, invades noncentromeric regions of the chromosome. These sites attract kinetochore proteins, which can become new centromeres and attach to spindle microtubules, resulting in chromosome breakage during mitosis. Consequently, understanding what features of CenH3 nucleosomes attract kinetochore proteins and how CenH3 location is strictly regulated remain key questions in chromosome biology.
Altered centromeric nucleosomal organization
Observations of the visually distinctive constriction on the chromosome by light microscopy have led to the hypothesis that the molecular structure of the centromere may be unusual. However, the high-resolution structure underlying centromeric chromatin has been elusive. In the past few years, using innovative experimental approaches in the budding yeast Saccharomyces cerevisiae and in cultured fruit fly and human cells, new studies have tested this model to reveal that the centromere is indeed unique, even at the level of individual CenH3 nucleosomes.
Normal nucleosomes are rigidly canonical in their organization, invariant across all the diverse eukaryotic organisms. As mentioned previously, they contain two copies of each histone, H2A, H2B, H3, and H4, wrapping 147 bp of DNA around the left-handed octameric protein core (Fig. 2a). However, when the yeast CenH3 is mixed in equal amounts with H2A, H2B, and H4 in a test tube with a kinetochore protein called Scm3, the result is an unprecedented hexameric nucleosome that has displaced one copy of H2A and H2B (Fig. 2b). When Scm3 is depleted from living cells, yeast CenH3 fails to associate stably with the native centromeric DNA, suggesting that this unusual CenH3 nucleosome is essential for normal centromere function in yeast.
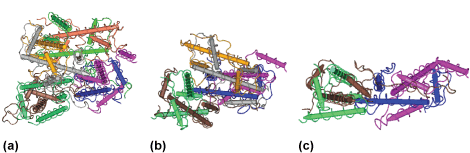
Topological (configuration) assays are very useful in assessing structural changes in nucleosomes, because the wrapping of DNA around histone complexes is spontaneous and dictated by the normally left-handed, positively charged histone ramp. Such studies have revealed that the curvature of DNA around the yeast CenH3 nucleosome follows a right-handed ramp rather than the canonical left-handed one. In the test tube, fruit fly CenH3 mixed with H2A, H2B, H4, and DNA also yields nucleosomes that wrap DNA in a right-handed ramp rather than the canonical left-handed ramp. The observation of oppositely wrapped DNA in both the yeast and fruit fly CenH3 nucleosomes makes a compelling argument for an unusual structure and suggests that this motif is a unique feature of CenH3 nucleosomes, regardless of which organism they are derived from.
Direct evidence for the alternative CenH3 nucleosome hypothesis comes from cultured cells from the fruit fly. In this case, each individual CenH3 nucleosome contains an equal amount of CenH3, H2A, H2B, and H4, cross-links to a tetramer form (rather than a stable octamer), and can be directly visualized as beads measuring one-half of the height of the canonical nucleosomes (Fig. 2c).
These studies thus provide the first indication of a histone variant–associated nucleosome that has, in all species studied so far, the centromere with a strikingly alternative nucleosomal structure, referred to as the hemisome, because all its properties are consistent with essentially half of an octameric nucleosome.
Alternative nucleosomal structure and epigenetic inheritance
What purpose could a tetrameric CenH3 nucleosomal structure serve? During DNA replication, when the two parental strands separate, the old nucleosomes are disassembled and must assemble anew on both daughter molecules in equal amounts. New canonical H3 nucleosomes reassemble behind the replication enzymes, providing a full complement of nucleosomes to both daughter DNA molecules. Surprisingly, unlike canonical H3, new CenH3 is added to the chromosome not at the time of DNA replication, but at the end of mitosis. This timing is particularly problematic because, although the DNA at the centromere is replicated together with the rest of the chromosome, its packaging information is essentially halved. Indeed, no new CenH3 nucleosomes would be available until the end of the next cell cycle to fill up the centromere. During replication, if CenH3 nucleosomes were to segregate randomly, one could envisage a scenario in which one daughter DNA could potentially receive more CenH3 nucleosomes than the other. In addition, CenH3 nucleosomes would be diluted by addition of H3 nucleosomes to gaps in the centromere created by replication, resulting in loss of integrity of centromere domains. Both of these events could then result in loss of chromosomes.
An elegant solution to this problem comes from the novel nucleosomal model for the centromeric nucleosome referred to above (Fig. 2c). During G2 phase, recently assembled CenH3 octamers are envisioned to be intrinsically associated, and would immediately revert into stable tetrameric halves (or hemisomes) during replication, thereby allowing both daughters to get equal numbers of CenH3 nucleosomes (Fig. 3). Because no new CenH3 would be assembled until after the next mitosis was completed, these tetramer nucleosomes effectively serve as the sole functional form during mitosis. This solution becomes particularly relevant for organisms, such as the budding yeast, that have only one centromeric nucleosome that must be shared between two daughter DNA molecules after replication, and to ensure that each daughter has a functional centromere ready for the next mitosis. This model allows for precise and equal segregation of the sole centromere-identifying epigenetic feature to daughter chromosomes after replication, and therefore provides an attractive solution for the epigenetic inheritance of centromeres.
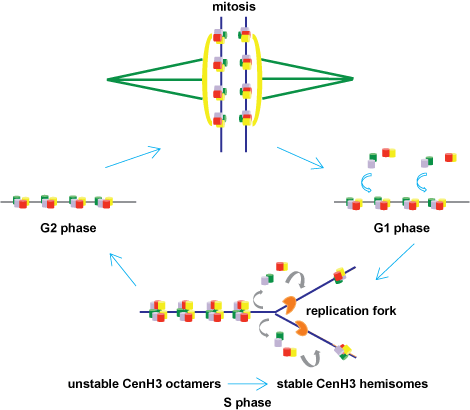
The hemisome model also posits a speculative scenario regarding the evolution of chromatin structure. The Archaea (a group of diverse microscopic prokaryotic organisms, including thermophiles and methanogens) are the earliest organisms known to have developed chromatin structure. The properties associated with eukaryotic CenH3 nucleosomes described above are similar to those seen in the archaeal domain of life. It has been proposed that the Eukaryotae and Archaea share the origins of nucleosomes from a common ancestor predating the split in the evolutionary tree between these two domains. The problem with this scenario has been that (1) archaeal nucleosomes discovered so far are highly flexible tetramers, with 80–120 bp of right-handed wrapped DNA, whereas all eukaryotic nucleosomes are rigid octamers with 147 bp of left-handed wrapped DNA; (2) the histone proteins between these two kingdoms are dissimilar; and (3) there are no known organisms that contain an intermediate transition form. However, the finding that eukaryotic CenH3 nucleosomes and archaeal nucleosomes both form tetramers and that both wrap DNA around a right-handed ramp is remarkable. This shared structural identity may derive from a proto-eukaryotic ancestral nucleosome that was co-opted for mitosis in early eukaryotes and evolved separately into the octameric packaging form needed for genome organization.
Future outlook
Observations from recent studies of centromeres have expanded our conceptual framework for understanding how the centromere is organized, how it is assembled, and how it may have evolved. As is the case for all novel concepts, more work must be performed to understand how dynamic biological processes and intrinsic biophysical properties contribute to the alternative CenH3 nucleosomal properties observed thus far, as well as to understand the functional consequences that such nucleosomes have on centromere function. Undoubtedly, ongoing studies and new methodologies will yield exciting and deep insights into these questions aimed at the very heart of eukaryotic biology.
See also: Archaea; Cancer (medicine); Chromosome; Deoxyribonucleic acid (DNA); Eukaryotae; Gene; Genetics; Mitosis; Nucleosome