Key Concepts
The science of biological inheritance. Genetics is responsible for the resemblances and differences among related organisms and individuals. As such, it occupies a central position in biology, for essentially the same principles apply to all animals and plants. Moreover, understanding of inheritance (see illustration) is basic for the study of evolution and for the improvement of cultivated plants and domestic animals. Genetics also may be defined as the science that deals with the nature and behavior of genes, which are the fundamental hereditary units. From this point of view, evolution is seen as the study of changes in the gene composition of populations, whereas embryology is the study of the effects of genes on the development of an organism. In general, genetics has much to contribute to the study of developmental biology, biochemistry, pathology, anthropology, and other subjects. See also: Animal evolution; Anthropology; Biochemistry; Developmental biology; Embryology; Evolution; Macroevolution; Pathology; Physical anthropology; Plant evolution; Population genetics
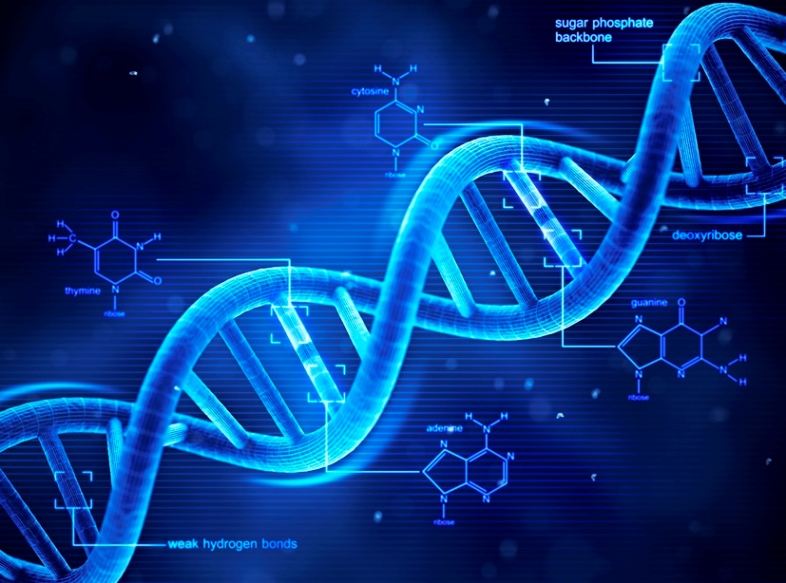
Study and analysis
The geneticist uses various methods of study, nearly all of which rest on the presence of differences between individuals. These techniques do not make it possible to analyze the portions of the hereditary makeup of individuals for which differences cannot be found. However, if differences occur, it is necessary that they be transmitted to later generations via sexual reproduction or some analogous process that allows the recombination of inherited properties from different individuals. In brief, what the geneticist usually does is to cross diverse individuals and study the descendants (or analyze existing pedigrees). Such a study must usually be carried through at least two successive generations and requires enough individuals to establish ratios between the classes present. The mechanism of heredity is such that its analysis requires the use of probability theory, but only very simple statistical concepts are typically needed. See also: Animal breeding; Biometrics; Heterosis; Human genetics; Mendelism; Plant breeding; Probability; Statistics
Molecular genetics
The field of molecular genetics describes the basis of inheritance at the molecular level. The genetic material in most organisms is deoxyribonucleic acid (DNA) [see illustration], which is a very long polymer made up of four different nucleotide monomers: adenine (A), guanine (G), cytosine (C), and thymine (T). A landmark discovery was the demonstration in 1953 by James Watson and Francis Crick that the genetic material consists of two DNA strands wrapped around each other to form a double helix. The two strands are held together by specific noncovalent, weak interactions between the nucleotides: A always binds to T, and G always binds to C. These long, double-stranded DNA molecules make up chromosomes, which are divided into thousands of different functional units called genes. The genes specify the structure and function of an organism. See also: Allele; Chromosome; Deoxyribonucleic acid (DNA); Gene; Nucleic acid; Nucleotide
Typically, molecular geneticists focus on two general questions: how do genes specify the structure and function of organisms, and how are genes replicated and transmitted to successive generations? Both questions have been answered. Genes specify organismal structure and function according to a process described by the central dogma of molecular biology: DNA is made into ribonucleic acid (RNA), which specifies the structure of a protein (a polymer of different amino acids) that carries out a particular function. In the first step of gene expression, DNA is transcribed into messenger RNA (mRNA)—a single-stranded polymer of nucleotides very similar to DNA—by the enzyme RNA polymerase. In the second step, the mRNA molecule serves as a template for protein synthesis, which is carried out by a complex machinery that comprises a particle called a ribosome and special adapter RNA molecules called transfer RNAs (tRNAs). See also: Amino acid; Molecular biology; Protein; Ribonucleic acid (RNA); Ribosomes
The specific sequence of nucleotides (A, T, G, and C) in the DNA specifies gene function in two ways. First, particular sequences signal the RNA polymerase to begin and end synthesis of the mRNA. In addition, specific DNA sequences ensure that RNA polymerase transcribes certain genes only in the correct cell type at the proper time. A major goal of molecular genetics is to learn how DNA sequence determines the regulation of gene expression. The second way in which the nucleotide sequence specifies gene function is by determining the structure of the encoded protein. The sequence of the mRNA is read by the ribosome in groups of three nucleotides, each of which is called a codon. The four different nucleotides are arranged in 64 codons that specify the 20 amino acids in proteins. Therefore, the DNA sequence determines the structure and function of an organism. Molecular geneticists have learned how to determine large amounts of DNA sequence with relative ease. Large-scale projects have determined the complete DNA sequence of several organisms, including humans. Although it is clear how the sequence of DNA determines the sequence of protein, a major challenge remaining for molecular geneticists is to explain how the sequence of a protein determines its particular function. See also: DNA sequencing; Genetic code; Genetic mapping; Human genome
Overall, the structure of DNA provides a simple mechanism for genes to be faithfully reproduced. The specific interaction between the nucleotides means that each strand of the double helix carries the information for producing the other strand. This duplication process is accomplished by several enzymes, including DNA polymerase. It copies each DNA strand into two molecules of double-stranded DNA that are apportioned to each new cell during cell division. Rarely, DNA polymerase makes a mistake during replication, or the DNA becomes damaged, and an altered gene results. Such mutations can change the function of the encoded protein, thereby leading to evolutionary change. In addition, DNA molecules can recombine through complex processes that involve the breakage and rejoining of two molecules and lead to different gene combinations that can alter the structure and function of the organism. See also: Genetic engineering; Mutation; Recombination (genetics)