Superposition is a confounding yet foundational principle of quantum physics in which a quantum system—for example, a group of subatomic particles or atoms—can exist in multiple quantum states simultaneously. For example, consider a coin that is both heads- and tails-side-up until it is flipped and observed, whereupon the coin falls into one of the two possible states. To date, researchers have observed this strange quantum behavior almost exclusively in small-scale systems, where quantum effects readily emerge. Now, for the first time, scientists have physically demonstrated the superposition principle with large molecules consisting of nearly 2000 atoms. In an experiment reported in September 2019, these molecules acted like particles and waves simultaneously—another odd feature of quantum mechanics related to superposition—and interacted such that they created wave interference patterns, which are indicative of wavelike behavior. The patterns that the molecules formed were similar to those generated when ripples on a pond intermingle, yielding larger-amplitude waves in which crests or troughs double-up, as well as smaller waves in which crests and troughs overlap. See also: Atom; Interference of waves; Molecule; Quantum mechanics; Superposition principle; Wave (physics)
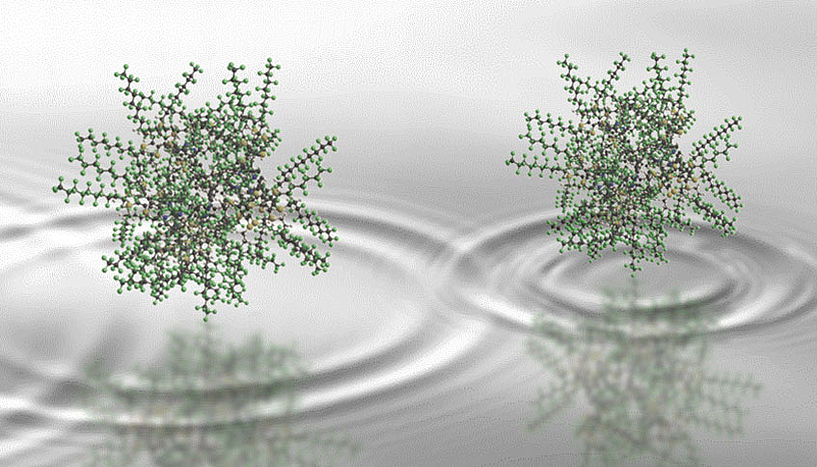
Superposition had previously been deeply borne out for subatomic particles such as photons and electrons, as well as on the larger scale of atoms and even into the range of small molecules. The new demonstration, though, involved molecules with around 25,000 atomic mass units—about two orders of magnitude greater than those recordholder molecules. (An atomic mass unit is equal to exactly 1/12 of the mass of an atom of carbon-12.) Researchers custom-synthesized the molecules—a kind of porphyrin, or cyclic carbon-based compound—so that scientists could beam the molecules into an interferometer apparatus whose detectors ultimately registered interference patterns. See also: Atomic mass unit; Interferometry; Porphyrin
These unprecedented results offer insight into the boundary between the infinitesimal realm of quantum physics and the everyday human realm of classical physics. In the former, objects behave probabilistically and exist across a range of values; in the latter, objects have definite locations and properties. An enduring question in science is what exactly upholds the apparent transitional dichotomy between quantum and classical physics. At a minimum, these new results expand the mass range within which quantum effects can still apparently manifest. Beyond that, the results also lend support to models that attempt to bridge the quantum-classical divide. These models start with the wave function of a particle, which describes its quantum mechanical propensity to possess a range of values. The wave function is said to collapse upon measurement to a single, discrete value, such as for a particle's location, momentum, or energy. The models that serve to reconcile quantum and classical interpretations of the world suggest that the rate of a particle's wave-function collapse is proportional to the square of the particle's mass, thus relegating heavier particles (or the objects they comprise) to increasingly definite, classical-like states. Further advances in producing wave-particle duality in ever-more-massive molecules will hone this approach at unification. See also: Classical mechanics; Quantum statistics; Wave equation quantum superposition; quantum physics; quantum theory; quantum mechanics; quantum world; classical world; classical physics; superposition