Key Concepts
A system of chemical communication among cells in vertebrate animals. The classical vertebrate endocrine system (Fig. 1) consists of a group of discrete glands that secrete unique products (hormones) into the bloodstream. These products travel in the blood to distant sites or targets, where they cause specific physiological responses. Thus, endocrine glands differ from exocrine glands because they lack ducts and deliver their secretions in the bloodstream. The classical definition of an endocrine system is also harder to apply with the discovery of scattered cells rather than discrete glands that act as endocrine organs, of endocrine cells that affect themselves (autocrine effect) or nearby targets (paracrine effect) by diffusion through extracellular fluids rather than the bloodstream, and of neurons that secrete hormones (neurosecretion). All of these mechanisms, however, allow for chemical intercellular communication and can be considered part of the endocrine system. See also: Circulation; Endocrine mechanisms; Endocrinology; Gland; Hormone; Neurosecretion; Vertebrata
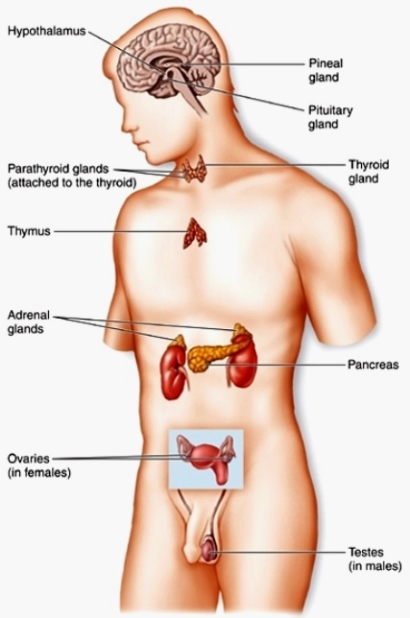
Homeostasis
One important function of the endocrine system, along with the nervous system, is to maintain homeostasis, that is, a constancy of the internal environment of an organism. Thus, an organism reacts and adjusts physiologically to changes in its external environment. For example, certain species of fish migrate from freshwater to seawater, or vice versa, and adjust to changes in salt concentrations by endocrine and neuronal responses. The roles of the endocrine and nervous systems in maintaining homeostasis are many, complementary, and overlapping. See also: Homeostasis; Nervous system (vertebrate)
Nature of hormones
Hormones are the products of endocrine cells. They are either proteinlike (amino acids, peptides, proteins, and glycoproteins) or steroidal. Peptide hormones (Fig. 2) are produced by protein synthetic mechanisms directed by the genes of the endocrine cells. Peptide hormones are usually produced as precursors (prohormones). In some cases, two or more different hormones are enclosed within the larger prohormone. These prohormones are cleaved to the smaller peptide hormone or hormones just before leaving the endocrine cell or when they reach their target. One reason that prohormones are produced may be that they are biologically inactive and thus do not cause physiological changes when stored. Protein hormones are stored in endocrine cells in secretory granules that bud off the endoplasmic reticulum and Golgi membranes, where protein synthesis occurs. The granules leave the cell by endocytosis and enter the bloodstream. See also: Endoplasmic reticulum; Golgi apparatus; Protein; Steroid
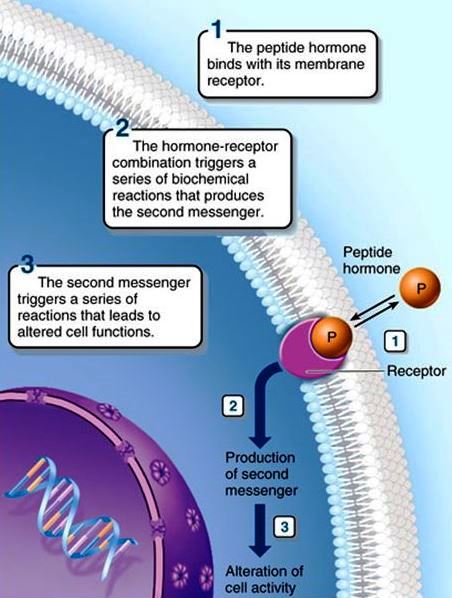
On the other hand, steroid hormones are produced from cholesterol by a number of well-characterized, enzyme-catalyzed steps. Cholesterol is thus converted stepwise to various steroid families—the hormones of the adrenal cortex (cortisol and aldosterone), the estrogens (estradiol) from the ovary, and the androgens (testosterone) from the testis. Steroid hormones diffuse across the endocrine cell plasma membrane to enter the circulation. See also: Adrenal gland; Androgens; Cell membrane; Cholesterol; Estrogen
Receptors
Hormones cause physiological responses in their targets. Because, in most cases, the blood carries hormones throughout the body, there must be a system by which only certain tissues respond to each hormone. This is accomplished by receptors, which are binding sites either on the surface of the target cell or within its nucleus. Receptors are high-molecular-weight proteins; the structure of some, such as the insulin receptor, is known. In general, peptide hormones cannot cross the plasma membrane, so their receptors are located there. In contrast, steroid hormones do pass through the plasma membrane of their targets and bind to nuclear receptors, probably located in the deoxyribonucleic acid (DNA). See also: Deoxyribonucleic acid (DNA)
Second messengers
In order for peptide hormones to stimulate physiological changes within the target cell, the "message" must be passed from the hormone–receptor complex of the plasma membrane to the interior of the cell. This process of signaling across the plasma membrane is accomplished by so-called second messengers; one of the best-known second messengers is adenosine 3′,5′-cyclic monophosphate (cyclic AMP). The breakdown of cell-membrane phospholipids in response to hormone–receptor interaction is another widespread mechanism of transmembrane signaling that mobilizes calcium ions within target cells. Calcium ions, in turn, are important second messengers. Steroid hormones, of course, have no need for second messengers because they are lipid-soluble and pass readily through the plasma membrane and into the cell. See also: Lipid; Phospholipid
Physiological responses
Once hormones are bound with their receptors and have stimulated their target cells, physiological responses occur. This may involve a variety of biochemical processes, including conversion of an inactive form of an enzyme into an active one, stimulation of critical enzymatic pathways, increased transport of glucose or amino acids into cells, or synthesis of new proteins. These events may result in overall changes in cell or organ function, metabolism, growth, or even behavior of the organism. See also: Enzyme
Feedback systems
The endocrine system is regulated by control mechanisms, which are the means by which homeostasis is achieved. The most common relationship between a hormone and its target is one of negative feedback, whereby the response to the hormonal stimulus turns off the original stimulus. For example, the endocrine pancreatic beta cells produce insulin in response to high blood sugar levels. Insulin is released into the blood, where it causes its target cells to take up glucose, thus reducing blood sugar. When blood glucose concentration falls, the secretion of insulin is turned off. The system is turned back on by the following process: when less insulin is secreted, the target cells take up less glucose, and thus the blood glucose content gradually increases again until insulin secretion is stimulated once more. Therefore, the blood level of the hormone (insulin) and the substance (glucose) responding to the hormone are kept within normal ranges. See also: Carbohydrate metabolism; Glucose; Insulin
Most relationships between hormones and their targets involve negative feedback. However, positive feedback also occurs occasionally, whereby the production of the hormone is stimulated rather than turned off by the product of the hormone–target interaction.
Pituitary gland and hypothalamus
The pituitary gland, or hypophysis, is located near the base of the brain. It secretes many hormones (Fig. 3) and controls the function of other endocrine glands. The production and release of the various pituitary hormones are regulated in turn by small peptide-releasing hormones from the hypothalamus of the brain. These factors are produced by neurosecretory neurons and travel to the adenohypophysis (anterior lobe of the pituitary) by way of a portal blood system. The releasing hormones stimulate specific cells of the adenohypophysis to produce and release their hormones. Generally speaking, each of the adenohypophysial hormones is affected by a separate releasing hormone. For example, the hypothalamic thyrotropin-releasing hormone stimulates the synthesis and release of thyroid-stimulating hormone (thyrotropin) by the adenohypophysis. See also: Brain; Pituitary gland
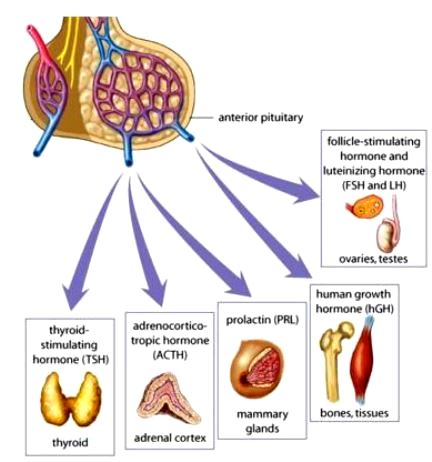
Other adenohypophysial hormones include adrenocorticotropic hormone, which stimulates the production of steroid hormones by the adrenal cortex; growth hormone, which stimulates protein synthesis and growth in many cells; prolactin, which stimulates the production of milk by the mammary glands and is important in salt and water balance and many other functions; follicle-stimulating hormone, which induces growth of the follicles of the ovary prior to ovulation; and luteinizing hormone, which induces ovulation in the ovary. The release of both follicle-stimulating and luteinizing hormones is governed by gonadotropin-releasing hormone.
Other hypothalamic hormones do not reach the pituitary by way of the bloodstream; instead, they travel down the long axons of neurosecretory cells into the neurohypophysis (posterior lobe of the pituitary). These hormones—oxytocin and vasopressin (an antidiuretic hormone)—are released directly from the axon end bulbs into the blood. Oxytocin acts upon the mammary glands of female mammals to cause milk release in response to suckling by the young, and stimulates the uterus to contract at the end of pregnancy to aid in expulsion of the offspring. Vasopressin is important in water conservation (less urine excretion) by the kidney tubules and also produces an increase in blood pressure.
Thyroid gland
The thyroid gland lies in the neck region of mammals. It produces two closely related hormones—triiodothyronine and thyroxine. Both of these hormones increase the metabolic rate of an organism, and they increase enzyme activity and protein synthesis. The thyroid hormones act along with growth hormone to promote cell growth and development. Thyroid hormones are peptides, but their three-dimensional structures may be similar to those of steroid hormones. Thus, they are unusual in their ability to pass through the plasma membrane of their target cells and bind to nuclear receptors, directly affecting genes that control protein synthesis. See also: Thyroid gland; Thyroid hormones
The control of hormone secretion by the thyroid, as well as by the adrenal cortex and gonads, involves more complex feedback relationships. These endocrine glands are affected by the levels of hormones from the adenohypophysis (note that the hormones are also affected by releasing hormones from the hypothalamus). In the case of the thyroid gland, thyrotropin-releasing factor from the hypothalamus stimulates the release of thyroid-stimulating hormone by the adenohypophysis. In response, the thyroid secretes thyroxine and triiodothyronine. High blood levels of the thyroid hormones inhibit the secretion of both thyrotropin-releasing factor (long-loop feedback) and thyroid-stimulating hormone (short-loop feedback).
Calcium regulation
The parathyroid glands derive their name from the fact that, in mammals, they are embedded within the thyroids. These small glands are essential for life because they regulate the concentration of calcium ions in blood and other extracellular fluids. If calcium is too low, the animal goes into tetanic convulsions and dies; in contrast, if calcium is too high, abnormal calcification and stone formation can occur. Parathyroid hormone is a protein hormone that raises the blood calcium levels (hypercalcemia). The hormone acts upon bone to cause the release of calcium and phosphate, and upon the kidney to increase the reabsorption (conservation) of calcium and the excretion of phosphate. See also: Bone; Calcium; Calcium metabolism; Kidney; Parathyroid gland; Parathyroid hormone
Vitamin D is recognized as a steroidlike hormone, although it does not originate from an endocrine gland. It is synthesized from precursors present in the diet or produced after exposure of skin lipids to ultraviolet light. Vitamin D plays roles in calcium conservation by the kidney and in bone mineralization, but its most important function is to enhance calcium transport across intestinal cells and thus conserve dietary calcium. See also: Vitamin D
Calcitonin (thyrocalcitonin) is another peptide hormone produced by thyroid cells in mammals and from the ultimobranchial glands of nonmammalian vertebrates. Calcitonin is hypocalcemic and acts by inhibiting calcium loss from bone. Of the three calcium-regulating hormones, it appears to be the least important. See also: Calcitonin; Ultimobranchial bodies
Carbohydrate regulation
Insulin and glucagon are peptide hormones produced by endocrine cells of the pancreatic islets. Insulin is a protein hormone produced by the pancreatic beta cells and is the only hormone that decreases blood sugar (glucose) levels. It acts on its target cells (skeletal muscle and fat cells) to increase the uptake of glucose, amino acids, and fatty acids. Once taken into cells, glucose is used in metabolic reactions or stored as glycogen (a large carbohydrate). Insulin also causes the conversion of amino acids to proteins and fatty acids to fats in the target cells. In the absence of insulin, as in diabetes mellitus, the target cells cannot take up glucose, and thus the body must utilize amino acids and fats as energy sources. These processes result in the accumulation of toxic metabolic products that eventually disrupt the acid–base balance of the body, leading to coma and death. See also: Amino acid; Diabetes; Energy metabolism; Fat and oil; Glucagon
Glucagon, in contrast, is a hyperglycemic hormone. It is a small peptide from the pancreatic islet alpha cells that acts upon liver cells to cause the conversion of glycogen to glucose by activation of key enzymes in a complex metabolic pathway.
In addition, many other hormones elevate blood sugar levels. For example, epinephrine (adrenalin), an amino acid derivative from the adrenal medulla, acts by the same pathway as glucagon to convert glycogen to glucose, except that the targets of epinephrine are skeletal and heart muscles. Epinephrine is secreted in times of stress and serves to prepare the body for an emergency by increasing the availability of energy in the form of glucose and by increasing the heart rate and blood pressure. See also: Epinephrine
Growth hormone (a large protein hormone from the adenohypophysis) is secreted in response to low blood sugar levels. This hormone elevates blood sugar by blocking the uptake of glucose by cells and by favoring the utilization of fats rather than glucose as an energy source.
Finally, many of the adrenal cortical hormones, such as cortisol, are known collectively as glucocorticoids because they also elevate blood glucose levels. These steroid hormones favor the production of glucose from proteins and fats rather than glycogen. Glucocorticoids also exert an anti-inflammatory action, which makes them useful for treatment of arthritis and other diseases.
Salt and water regulation
Several hormones affect the ability of the kidney to conserve or excrete salts and water. Vasopressin promotes water reabsorption by the kidney tubules, so the organism excretes less water. The secretion of vasopressin is regulated by hypothalamic neurosecretory neurons that are sensitive to the concentration of salts in extracellular fluids. In the absence of vasopressin, an individual excretes great volumes of dilute urine, leading to severe dehydration (diabetes insipidus). See also: Osmoregulatory mechanisms; Urine
Salt excretion is regulated mainly by two hormones that act in opposition. Aldosterone is an adrenal cortical steroid that promotes the reabsorption of sodium by the kidney tubules and thus decreases its excretion in the urine. In contrast, atriopeptin (atrial natriuretic factor, which is a peptide that originates in heart muscle) acts upon the kidney to increase the excretion of sodium in the urine. See also: Excretion
Reproductive hormones
Probably, the best-studied endocrine glands are the gonads, that is, the testes of the male and the ovaries of the female. The gonads are regulated by follicle-stimulating hormone and luteinizing hormone from the adenohypophysis. In both males and females, the sex hormones affect reproductive behavior. See also: Reproductive behavior; Reproductive system
In the male, follicle-stimulating hormone stimulates the initiation of sperm formation by the testis tubules, and luteinizing hormone acts on the nearby Leydig cells of the testis to produce testosterone (the principal male sex hormone). Testosterone acts by a paracrine mechanism to cause the final maturation of sperm, and by way of the blood to stimulate development of the male reproductive system and secondary sex characteristics (for example, body shape, beard, and muscle growth). See also: Testis
In the female, follicle-stimulating hormone stimulates the growth of the ovarian follicles at the beginning of each reproductive cycle. As the follicles grow, they produce estradiol, which is an important female sex hormone. Increasing levels of estradiol cause feedback inhibition of the released gonadotropin-releasing hormone. High levels of estradiol also have an unusual positive feedback effect upon the hypothalamus and adenohypophysis to cause a surge in the secretion of luteinizing hormone, which results in ovulation (the release of the ovum). The corpus luteum (a remnant of the ovulated follicle) produces both estradiol and progesterone. Progesterone is necessary for the maintenance of a quiescent uterus during pregnancy, and both estradiol and progesterone are important in the regulation of the female reproductive cycle (for example, the rhythmic timing of the menstrual cycle in primates). Estradiol is also essential for the growth and maturation of the female reproductive system and secondary sex characteristics (for example, breast growth, body shape, and patterns of fat deposition). See also: Ovary; Progesterone