In a potential watershed moment for physics, the first results from the Muon g-2 (pronounced gee minus two) experiment have strongly indicated that particles called muons deviate from precise predictions of their behavior in the standard model of particle physics. The findings, announced on April 7, 2021, suggest that undiscovered particles or forces in the universe are responsible for the muon's unexpected variance. See also: Physics; Standard model
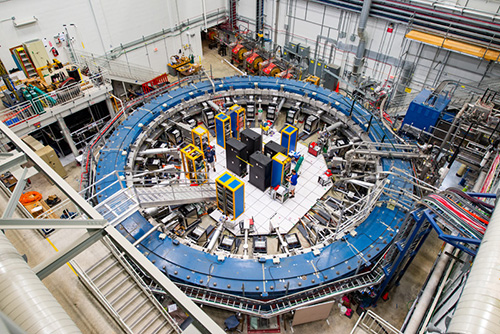
Researchers designed the Muon g-2 experiment to leverage particular properties of the muon that make this particle an ideal test of the rigorous, interrelated framework of the standard model. Developed over the past half-century and borne out by increasingly stringent experimentation, the standard model deeply explains three of nature's four fundamental interactions, or forces—namely, electromagnetism and the strong and weak nuclear interactions—using the laws of quantum mechanics. As one of nature's 17 known elementary particles in the standard model, muons are very similar to electrons. Both belong to the class of particles known as leptons and possess a negative electric charge, although the muon has about 200 times the electron's mass. As a charged particle, a muon acts as though it has an internal magnet. When exposed to an external magnetic field, the direction of a muon's magnet precesses, or wobbles, in a manner akin to the axis of a spun top. The strength of the particle's internal magnet determines the precession rate, given a magnetic field of a certain strength. See also: Electromagnetism; Electron; Elementary particle; Fundamental interactions; Lepton; Magnetism; Precession; Quantum mechanics; Strong nuclear interactions; Weak nuclear interactions
Using the standard model, physicists can precisely calculate a value—the g-factor—that describes this behavior of muons and other particles. Furthermore, the standard model allows for the calculation of an expected deviation in the g-factor caused by muons interacting with subatomic particles that constantly flit in and out of existence, which are known as virtual particles. This conversion of energy to matter and back to energy is thoroughly accounted for in the standard model. As muons move, they should encounter these virtual particles. That interaction alters the g-factor slightly, incurring what physicists refer to as an anomalous magnetic moment. Overall, both the g-factor and the anomalous magnetic moment for muons can be calculated to extreme precision, with the accepted theoretical values for each as 2.00233183620(86) and 0.00116591810(43), respectively (with the uncertainty of each prediction in parentheses). See also: Electron spin; Energy; Matter
Particle accelerators can generate muons in abundance, and for the Muon g-2 experiment—located at the U.S. Department of Energy’s Fermi National Accelerator Laboratory (Fermilab) near Chicago, Illinois—a beam of these particles is fed into a powerful superconducting magnetic storage ring about 15 meters (50 feet) in diameter. (That ring originally served as the main component of a similar experiment previously run at the Brookhaven National Laboratory in New York; the massive ring was transported by sea and then by ground to the Chicagoland area to take advantage of the powerful muon beam at Fermilab.) The Muon g-2 experiment measures the precession of the muons' magnetic moment as muons circulate in the ring and interact with virtual particles. The initial results, when combined with the Brookhaven data, overall do not agree with the standard model's figures, instead finding a g-factor of 2.00233184122(82) and an anomalous magnetic moment of 0.00116592061(41). These results have a statistical significance of 4.2 sigma; while they fall short of physicists' standard of five deviations to announce an unequivocal discovery, they nevertheless still have only 1-in-38,000 odds of being due to chance. The data reported so far are only 6% of the total that Muon g-2 is expected to ultimately collect, so scientists should know in a few years' time whether the standard model has, in fact, been broken. See also: Particle accelerator; Statistics; Superconducting devices