Key Concepts
The component of electromagnetism involving magnets, magnetic fields, and magnetic forces. Magnetism encompasses all phenomena relating to the magnetic field (Fig. 1) and its effects on other entities. Magnetism is part of electromagnetism, which is one of the fundamental interactions of the universe. Although a magnetic field is an inseparable component of the electromagnetic field, it can be treated as independent in some situations. Magnetism involves the interplay of four basic entities: electric currents, intrinsic magnetic dipole moments, magnetic fields, and electric fields. An electric current consists of electrically charged particles that are moving. An intrinsic magnetic dipole moment is an innate property of certain fundamental particles. Collections of electric currents and particles with intrinsic magnetic moments are known as magnets. See also: Electric charge; Electric current; Electromagnetism; Fundamental interactions; Magnet
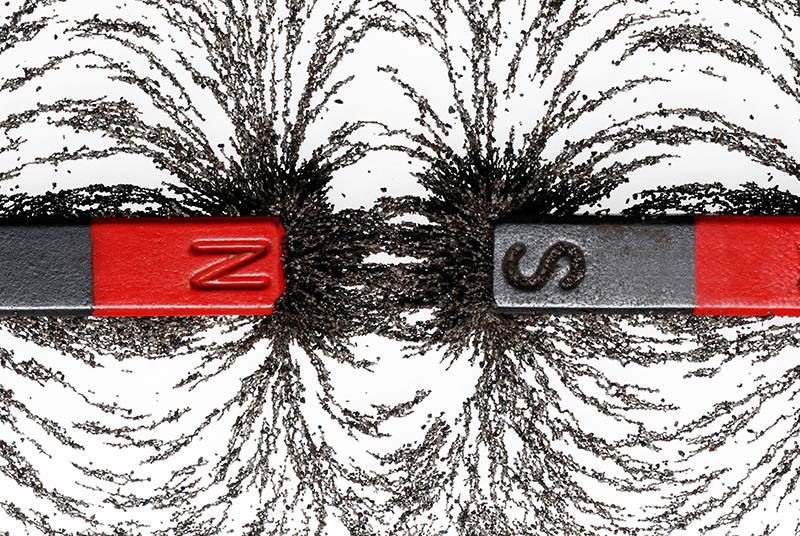
Magnetic moments
Any object that creates a magnetic field can be characterized as a magnetic moment. A magnetic moment is a particular configuration of north magnetic poles and south magnetic poles. The magnetic moment of an object determines the type and strength of magnetic field that it produces..
Magnetic monopoles
A magnetic monopole is a single, isolated, north magnetic pole or a single, isolated, south magnetic pole. There is presently no evidence that magnetic monopoles exist. The question of their existence is one of the great unsolved problems in physics. See also: Magnetic monopoles; Physics
Magnetic dipoles
A magnetic dipole is a north magnetic pole connected inseparably to a south magnetic pole. A magnetic dipole moment can either appear as an intrinsic property of a particle, such as an electron, or can arise from a simple loop of electric current, such as in an atom or in a coiled wire. A magnetic dipole moment acts like a small bar magnet. In addition to its intrinsic dipole moment, an atomic electron also has an orbital dipole moment that arises from its circulating motion in the atom. The total dipole moment of the electron is the sum of its intrinsic dipole moment and its orbital dipole moment. See also: Atom; Electron
Magnetic quadrupoles and other multipoles
As a consequence of there being no magnetic monopoles, higher-order magnetic moments must be collections of magnetic dipoles. This means that magnetic tripoles do not exist. A magnetic quadrupole consists of two antiparallel magnetic dipoles. (Note that two parallel magnetic dipoles act dominantly as a single dipole.) A magnetic sextupole consists of three magnetic dipoles, and so forth.
Magnets
A magnet is a macroscopic object that creates a magnetic field and therefore has a magnetic moment. Although most magnets are dipole magnets, higher-order magnets do exist. A magnet contains a collection of electric currents, such as the free currents in an electromagnet or the bound currents in a permanent magnet. Magnets also contain intrinsic dipole moments that can be treated mathematically as bound currents. The four types of magnets are permanent magnets, induced magnets, electromagnets, and induced electromagnets. See also: Magnet
Magnetic fields
A magnetic field is a vector field that extends through space and can interact with objects. The magnitude of the magnetic field vector at a point in space specifies the strength of the magnetic field at that location. The directionality of the magnetic field vector specifies the direction that a magnetic compass would point at that location. The magnetic field can be represented graphically using a magnetic field line diagram (Fig. 2). In such a diagram, magnetic field line arrows trace from north poles to south poles outside magnets and from south poles to north poles inside magnets. The arrows do so in such a way that the magnetic field lines always form closed loops. Although the magnetic field is an inseparable component of the electromagnetic field, the magnetic field can be treated as independent when it is static or changing slowly.
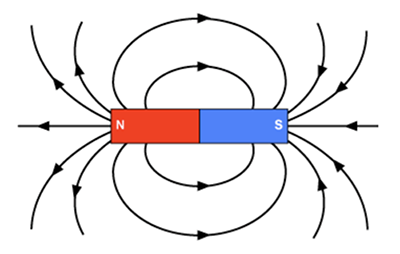
Effect of electric currents
Electric currents always produce magnetic fields. In electromagnets and induced electromagnets, the currents move freely through a conductor. In permanent magnets and induced magnets, the currents are bound in atoms and molecules and consist of circulating electrons. In the 1820s, scientists such as French physicists Jean-Baptiste Biot, Félix Savart, and Andre-Marie Ampère determined quantitatively how electric currents produce magnetic fields and exert magnetic forces. The differential equation summarizing how a current produces a magnetic field is known as Ampère’s law. When cast into a simplified integral form, Ampère’s law is known as the Biot-Savart law. See also: Ampère's law; Biot-Savart law
Effect of intrinsic moments
Intrinsic moments, such as that of an electron, always produce magnetic fields. Along with bound currents, intrinsic moments contribute to the magnetic field of permanent magnets and induced magnets. Because of its dependence on quantum spin, an intrinsic moment can be treated mathematically as a small loop of current. See also: Spin (quantum mechanics)
Effect of changing electric fields
A changing electric field produces a magnetic field. This principle is accounted for by adding an extra mathematical term to Ampère’s law, expanding it into the Ampère-Maxwell law. In practice, the magnetic field that is produced by a changing electric field is often insignificant. The most significant role of this effect is in enabling electromagnetic waves to exist and to be self-propagating. See also: Electromagnetic wave; Electromagnetic wave transmission
Magnetic forces
The magnetic field exerts a force on electric currents, intrinsic magnetic moments, and magnets (which contain collections of currents and intrinsic magnetic moments). Intrinsic magnetic moments can be treated mathematically as small loops of current. Therefore, all magnetic forces can be expressed as the interaction between the magnetic field and currents.
Magnetic force on a moving charged particle
The magnetic force law describes how a magnetic field B exerts a force F on a moving charged particle. This law is part of the Lorentz force law, which describes both electric and magnetic forces. The magnetic force law states:

Here q is the particle’s charge, ν is the particle’s velocity, and θ is the angle between the particle’s direction of motion and the direction of the magnetic field. This expression implies that a magnetic field exerts a force on a charged particle only when the particle is moving. This expression also shows that the force is largest when the magnetic field is perpendicular to the direction of motion of the charged particle, and there is no force if the field is parallel to the direction of motion. See also: Motion
The magnetic force law further states that the directionality of the magnetic force is always sideways relative to the motion of the particle. Therefore, the magnetic force can only change the direction of the particle but cannot accelerate it. As a result, a magnetic field can never do mechanical work directly. For a charged particle moving freely in space, the sideways nature of the magnetic force causes the particle to travel along a helical trajectory around the magnetic field lines. This effect can be used to trap free particles magnetically, such as occurs in Earth’s ionosphere and in nuclear fusion reactors. See also: Ionosphere; Nuclear fusion; Particle trap
Magnetic force between magnets
Because all magnets contain collections of currents, magnets exert forces on each other. In principle, the force between two magnets can be calculated using the magnetic force law. In practice, however, such calculations are complicated. For simple magnets, an easier rule can be used: Opposites attract and likes repel. This means that a north magnetic pole attracts a south magnetic pole. It also means that two north poles repel each other and, similarly, two south poles repel each other. Determining the location of the poles of an induced magnet or electromagnet involves additional rules. The force between magnets is the operating principle behind electric motors and audio speakers. See also: Loudspeaker; Motor
Material effects
For mathematical convenience, the total magnetic field B is often separated into the sum of two partial magnetic fields: the magnetizing field H and the magnetization M. Historically, these fields have been called many terms, such as flux density, magnetic induction, and magnetic polarization. Modern scientists refer to these fields by their letter names to avoid confusion. The H field is the magnetic field associated with free currents; that is, the currents in conductors and in free space. The M field is the magnetic field associated with bound currents; that is, the currents in magnetized materials. The three main types of magnetic materials are ferromagnetic, paramagnetic, and diamagnetic. See also: Diamagnetism; Ferromagnetism; Paramagnetism
Faraday’s law of induction
A changing magnetic field always produces an associated electric field. This induced electric field is able to generate currents in conductors. English physicist and chemist Michael Faraday discovered this law in the mid-nineteenth century, leading him to invent the generator. Faraday’s law is also the operating principle behind transformers, induced electromagnets, and inductors. See also: Conductor (electricity); Electromagnet; Generator; Inductor; Transformer
Electromagnetism
Magnetism is only one component of electromagnetism. Magnetism can be treated as independent only when its associated electric effects are negligible or decoupled. In 1855, Scottish physicist James Clerk Maxwell organized all of the laws of electricity and magnetism systematically into one complete set. Maxwell found that when the magnetic field varies rapidly in time, electromagnetic waves are created and the full laws of electromagnetism must be used. Currently, the most accurate theory of electromagnetism is the quantum version of Maxwell’s equations, known as quantum electrodynamics (QED). An ongoing area of research in magnetism is determining the source of the large-scale magnetic field of our galaxy. See also: Electricity; Maxwell's equations; Milky Way Galaxy; Quantum electrodynamics; Quantum mechanics