Key Concepts
The natural biological and chemical processes through which inorganic and organic nitrogen are interconverted. It includes the processes of ammonification, ammonia assimilation, nitrification, nitrate assimilation, nitrogen fixation, and denitrification (Fig. 1). See also: Biogeochemistry
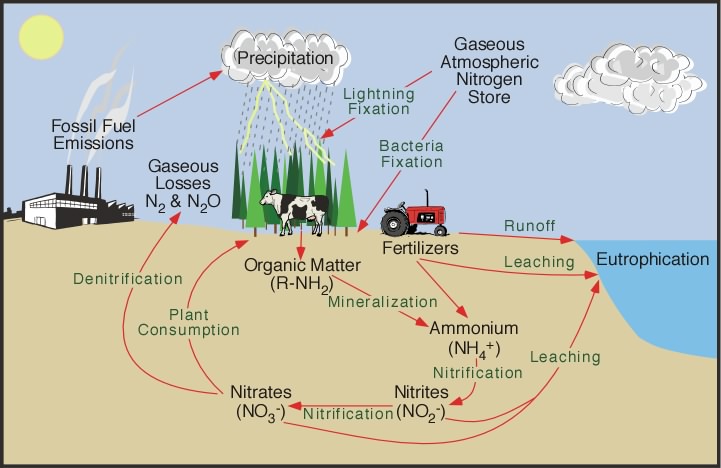
Nitrogen exists in nature in several inorganic compounds, namely N2, nitrous oxide (N2O), ammonia (NH3), nitrite (NO2−), and nitrate (NO3−), and in several organic compounds such as amino acids, nucleotides, amino sugars, and vitamins. In the biosphere, biological and chemical reactions continually occur in which these nitrogenous compounds are converted from one form to another. These interconversions are of great importance in maintaining soil fertility and in preventing pollution of soil and water. See also: Ammonia; Nitrogen oxides
Nitrogen reserves exist in five major sinks: the primary rocks, the sedimentary rocks, the deep-sea sediment, the atmosphere, and the soil-water pool. Although primary rocks contain as much as 97.8% of the Earth's total (1.9 × 1017 metric tons), their N2 contributes little to the nitrogen cycle. Of the remaining nitrogen, 2% is in the atmosphere as N2, and about 0.2% is in sedimentary rocks. The biosphere, consisting of the soil-water pool, contains only a small portion of the Earth's total nitrogen (2.4 × 1013 metric tons), and even here the predominant species (2.2 × 1013 metric tons) is N2 dissolved in the sea. In spite of this, it is in this soil-water pool that the major reactions of the nitrogen cycle occur. See also: Biosphere
An outline showing the general interconversions of nitrogenous compounds in the soil-water pool is presented in Fig. 1. The reactions are much more complex than in the outline, and biological agents have evolved intricate ways to manipulate these nitrogenous compounds for their own use. There are three primary reasons why organisms metabolize nitrogen compounds: (1) to use them as a nitrogen source, which means first converting them to NH3, (2) to use certain nitrogen compounds as an energy source such as in the oxidation of NH3 to NO2− and of NO2− to NO3−, and (3) to use certain nitrogen compounds (NO3−) as terminal electron acceptors under conditions where oxygen is either absent or in limited supply. The reactions and products involved in these three metabolically different pathways collectively make up the nitrogen cycle.
Three major microbial reactions contribute to the N2 cycle. First N2 is fixed (reduced) to ammonia, and the ammonia is used by N2-fixing microorganisms and by plants harboring N2-fixing microorganisms. Then these plants and bacteria, and animals and plants living off these plants and bacteria, die and lyse, and the nitrogen of their nitrogenous compounds is converted to ammonia by ammonification. The released ammonia is converted rapidly by nitrifying bacteria to NO3−, or it is used directly by microorganisms or plants. The NO3− produced by nitrification is used by plants and by bacteria as a nitrogen source; or if anaerobic conditions are created, the NO3− is denitrified (reduced) to N2 and N2O. This completes the nitrogen cycle.
Nitrogen compounds as nutrients
The synthesis of organic nitrogen compounds from inorganic nitrogen and carbon compounds begins with NH3 incorporation. One major reaction, catalyzed by glutamic acid dehydrogenase, involves 2-ketoglutarate, NADH (reduced nicotinamide adenine dinucleotide) and ammonia, and the product is glutamic acid. There are two ways in which organisms obtain ammonia. One is to use nitrogen already in a form easily metabolized to ammonia. Thus, nonviable plant, animal, and microbial residues in soil are enzymatically decomposed by a series of hydrolytic and other reactions to yield biosynthetic monomers such as amino acids and other small-molecular-weight nitrogenous compounds. These amino acids, purines, and pyrimidines are decomposed further to produce NH3 which is then used by plants and bacteria for biosynthesis, or these biosynthetic monomers can be used directly by some microorganisms. The decomposition process is called ammonification. Not all organic nitrogen is ammonified easily, and resistant nitrogenous residues constitute humus, a complex component of great importance to soil structure and water-holding capacity. See also: Amino acid; Biosynthesis; Catalysis and catalysts; Enzyme; Humus; Nicotinamide adenine dinucleotide (NAD); Soil
The second way in which inorganic nitrogen is made available to biological agents is by nitrogen fixation (this term is maintained even though N2 is now called dinitrogen), a process in which N2 is reduced to NH3. Since the vast majority of nitrogen is in the form of N2, nitrogen fixation obviously is essential to life. The N2-fixing process is confined to prokaryotes (certain photosynthetic and nonphotosynthetic bacteria). The major nitrogen fixers (called diazotrophs) are members of the genus Rhizobium, bacteria that are found in root nodules of leguminous plants (Fig. 2), and of the cyanobacteria (originally called blue-green algae). Even though rhizobia have been cultured so that they can fix nitrogen in the absence of the plant, the conditions needed for this fixation, such as a very low O2 concentration supplied by oxyleg-hemoglobin and the necessary carbon and energy sources supplied by the plant, are ideal in the nodule and are not easily met in the laboratory or elsewhere in nature. There are many “free-living” diazotrophs, and even though they appear to contribute little to soil nitrogen, most knowledge of the biochemistry of nitrogen fixation comes from studies of three of them, Clostridium pasteurianum, Azotobacter vinelandii, and Klebsiella pneumoniae. See also: Cyanobacteria; Nitrogen fixation; Prokaryote; Rhizosphere; Rhizosphere ecology; Root (botany); Soil microbiology
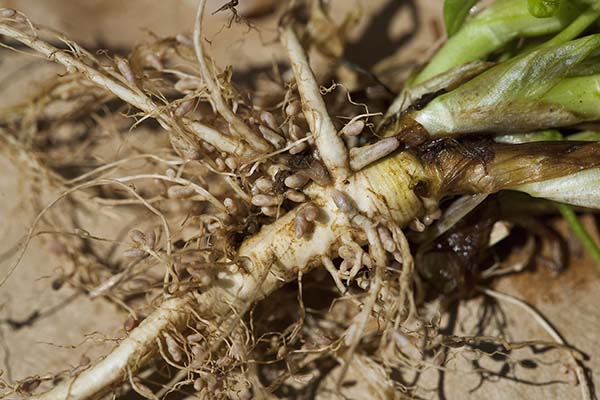
The nitrogen-fixing system of all these organisms is made up of two protein components. One, called the Fe protein, is a dimer of molecular weight about 60,000 daltons, and it contains an Fe4S4 center (similar to that of ferredoxin) that is involved in accepting and transferring the electrons needed for N2 reduction. It is the Fe protein that initially binds the magnesium adenosine triphosphate (MgATP) needed for N2 reduction. The second component, called the MoFe protein, is a tetramer of about 220,000 daltons and contains four Fe4S4 centers and two Fe8Mo1S6 centers, with the latter centers probably being the sites where N2 is reduced to NH3. For N2 reduction the MoFe protein accepts electrons from the Fe protein, and to facilitate the transfer, ATP is hydrolyzed to adenosine diphosphate (ADP) and inorganic phosphate. The N2 fixation process consumes as much as a third of the cell's energy supply, so for obvious reasons the N2-fixing system is not synthesized if a usable nitrogen compound other than N2 is available, that is, nitrogenase synthesis is repressed in the presence of NH3. See also: Adenosine triphosphate (ATP); Nitrogen fixation reactions
Nitrogen compounds as energy source
Many microorganisms can use organic nitrogen compounds as energy sources, but in most cases the nitrogen of the compound is first removed and excreted as NH3, and then the reduced carbon compound remaining is catabolized to yield both energy and organic carbon intermediates. A relatively few microorganisms (including some fungi) are able to aerobically convert the excreted NH3 to NO2−, and NO2− to NO3−, and couple these oxidations to the production of ATP and the creation of a membrane potential needed for biosynthetic reactions. Thus, NH3 added to aerobic soils is rapidly converted by such organisms to NO3−. Two chemoautotrophs (organisms that grow using an inorganic compound as an energy source and CO2 as a carbon source) are primarily responsible for NO3− production. First Nitrosomonas converts the NH3 to NO2−, and then the relatively toxic NO2− is rapidly oxidized to NO3− by Nitrobacter. Plants and microorganisms readily use this NO3− as a nitrogen source by first reducing it to NH3. The overall process of oxidation of NH3 to NO3− is called nitrification. The process whereby NO3− is reduced to NH3 is called nitrate assimilation. See also: Microbial ecology
Nitrogen compounds as electron acceptors
When NO3− accumulates in soils in which metabolizable carbon compounds are available and when such soils become anaerobic because growth of aerobic organisms exhausts the O2, certain organisms such as Pseudomonas, Micrococcus, Achromobacter, and Bacillus use the NO3− either as a normal electron acceptor or as an electron acceptor in place of O2. The electron acceptor is needed by such cells to allow electrons from cellular oxidations to flow through the array of electron carries in the cell membrane. This electron flow is needed to facilitate proton (H+) transfer across the membrane, which in turn creates a membrane potential and a pH gradient. The potential energy of the pH gradient is used in conjunction with the cells adenosine triphosphatase (ATPase) to allow ATP to be synthesized. The NO3− is the terminal electron acceptor in the electron flow and becomes reduced to NO2−, and the NO2− in turn is further reduced to N2 (some N2O may also be produced). The N2 (and some N2O) is released into the atmosphere. This process, called denitrification or nitrate respiration, is responsible for ridding many bodies of water and soil of excess fixed nitrogen that could lead to pollution by overproduction and decay of algae and other bacteria.
N2 cycle in the oceans
Most information on the N2 cycle comes from studies of the soil-water system. Much less is known about the nitrogen cycle in seas, even though it is estimated that as much as 20% of the N2 fixed on Earth occurs in the ocean. This N2 seems to be primarily fixed by cyanobacteria, although in localized areas some contribution by other photosynthetic and nonphotosynthetic bacteria also occurs. One calculation estimates that a single bloom of the cyanobacterium Trichodesmium could fix as much as 100 metric tons of N2 per day. The other processes associated with the nitrogen cycle, ammonification, nitrification, and denitrification, although obviously present in oceans, have been studied even less. Heterotrophic organisms were not shown to be responsible for nitrification in seas, and therefore chemoautotrophic marine nitrifiers, such as Nitrospira and Nitrococcus, appear to be the major nitrifiers in oceans. The denitrification step of the ocean's nitrogen cycle may account for a third of the Earth's total denitrification, but there is relatively little information on this. One suspects that most of this denitrification takes place in the anaerobic conditions of marine mud. See also: Marine microbiology