For the first time, researchers have used the spin rates of black holes in the wide-ranging and ongoing search for dark matter. A recent study proposed that if a certain kind of dark matter particle, known as an ultralight boson, existed in a particular mass range, the particles would collectively slow the spin rates of black holes. The black hole spin rates measured, however, proved twice as fast as the theoretical framework allowed, thus ruling out ultralight bosons in the observed mass range. This negative result helpfully narrows down the possible properties dark matter might possess, while also demonstrating a new kind of probe for subsequent searches in new black hole mass regimes. See also: Black hole; Mass
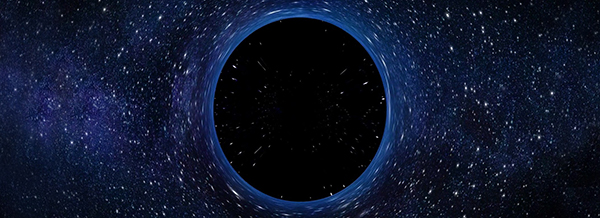
Dark matter is a puzzling substance outnumbering the "ordinary" matter in our universe five times over. Researchers have been developing theories about dark matter for about ninety years to account for the substantial, unseen, gravity-generating mass necessary to prevent stars from flying out of galaxies, as well as other unexplained astronomical observations. The scientific consensus is that dark matter consists of one or more undiscovered particles that may only interact with ordinary matter through the force of gravity. Yet many theories suggest that dark matter particles should occasionally annihilate each other or decay— producing detectable, known particles described in the standard model of particle physics that encompasses ordinary matter. Therefore, a tremendous number of experimental searches for dark matter have taken place to date. All of these searches, however, have failed to turn up direct evidence of dark matter. See also: Dark matter; Elementary particle; Gravity; Matter; Standard model
Physicists at the Massachusetts Institute of Technology (MIT) recently tried a new tactic for detecting dark matter which relies on the spin rates of black holes. When black holes merge, the event generates gravitational radiation, which manifests as wave-like deformations in the spacetime fabric of the universe that propagate outward like ripples across a pond. These waves pass clear through Earth, but in the process, they create miniscule displacements—on the order of ten-thousandth the diameter of a proton—that highly aligned systems of lasers can register. The measurable properties of the displacing waves in turn could reveal the masses and spins of the merging black holes that spawned these waves. A previously postulated quantum mechanical effect, called superradiance, holds that ultralight bosons could form in the space near a black hole. If ultralight bosons were indeed to form, these particles would collectively drag on the black hole and reduce its spin rate. See also: Gravitational radiation; Quantum mechanics; Spacetime
The MIT researchers reviewed the masses and spin rates of 45 black hole pairs cataloged by the Laser Interferometer Gravitational-wave Observatory (LIGO) in the United States and a second gravitational wave detector in Italy, called Virgo. The black holes in these pairs contained anywhere from 10 to 70 times the mass of the Sun. For ultralight bosons to form and measurably slow this set of black holes, according to superradiance, ultralight bosons would have to possess infinitesimal masses ranging from 1x10-13 electronvolts to 2x10-11 electronvolts, or about one-quintillionth (10-18) of an electron. Two of the black holes, though, had very high spin rates, eliminating ultralight bosons as dark matter candidates in this specific mass range. Nevertheless, because the theoretical mass that ultralight bosons could plausibly possess scales across 27 orders of magnitude, from 1x10-33 electronvolts to 1x10-6 electronvolts, only a tiny sliver of their full mass range has been excluded from consideration. The MIT study’s results also do not rule out heavier dark matter constituents, such as weakly interacting massive particles, or WIMPS, a long-favored candidate in the continuing hunt for dark matter. As LIGO and Virgo continue to gather gravitational wave observations, researchers plan to look for signatures of ultralight bosons with different masses. See also: Electron; Electronvolt; LIGO (Laser Interferometer Gravitational-wave Observatory); Weakly interacting massive particle (WIMP)