Key Concepts
The set of two successive cell divisions that serve to separate homologous chromosome pairs and thus reduce the total number of chromosomes by half. The process of meiosis (Fig. 1) includes two sequential nuclear divisions (meiosis I and II) that must occur prior to the formation of gametes [sperm and eggs (ova)]. The major purpose of meiosis is the precise reduction in the number of chromosomes by one-half, so a diploid cell (diploid = having two complete chromosome pairs in a nucleus) can create haploid gametes (haploid = having half of the diploid or full complement of chromosomes). To accomplish this reduction, a single parent cell undergoes two meiotic divisions to produce four daughter cells, with each having half of the original chromosome complement. The nonmeiotic (or somatic) cells of humans, for instance, have 46 individual chromosomes, or 23 pairs of homologous chromosomes. However, following meiosis, human eggs or sperm have only 23 chromosomes (one member of each pair). Reducing the number of chromosomes in the gametes to 23 allows the fusion of an egg with a sperm (in a process called fertilization) to result in an embryo with the requisite 46 chromosomes. Therefore, meiosis is a critical component of sexual reproduction. See also: Animal reproduction; Cell (biology); Cell biology; Cell division; Chromosome; Fertilization (animal); Fertilization (plant); Gametogenesis; Genetics; Ovum; Plant reproduction; Sperm cell
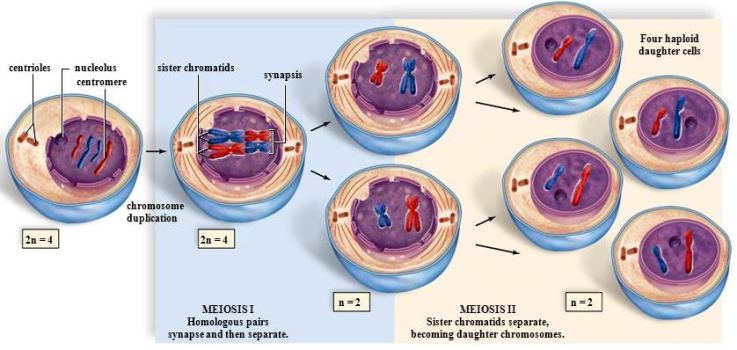
Chromosome behavior
For example, consider an organism that contains only two pairs of chromosomes. The chromosomes in each of these pairs are referred to individually as homologs; one is derived from the father of the organism and the other is derived from the mother. Both homologs carry the same array of genes. As the cell begins meiosis, each chromosome has already duplicated its deoxyribonucleic acid (DNA) and carries two identical copies of the DNA molecule. These are visible as two lateral parts, called sister chromatids, which are connected by a centromere. See also: Deoxyribonucleic acid (DNA); Role of telomeres and centromeres in meiotic chromosome pairing; Sister chromatid cohesion
The basic events of meiosis are actually quite simple. Homologous pairs of chromosomes are first identified and matched. This process, which occurs only in the first of the two meiotic divisions, is called pairing. The matched pairs are then physically interlocked by recombination, which is also known as exchange or crossing-over. After recombination, the homologous chromosomes separate from each other; then, at the first meiotic division, they are partitioned into different nuclei. The second meiotic division begins with half of the original number of chromosomes. During this second meiotic division, the sister chromatids of each chromosome separate and migrate to different daughter cells.
The patterns by which genes are inherited are determined by the movement of the chromosomes during the two meiotic divisions. It is a fundamental tenet of Mendelian inheritance that each individual carries two copies of each gene—one derived from its father and one from its mother. Moreover, each of that individual's gametes will carry only one copy of that gene, which is chosen at random. The process by which the two copies of a given gene are distributed into separate gametes is referred to as segregation. Thus, if an individual is heterozygous at the A gene for two different alleles (alternative forms of a gene), A and a, his or her gametes will be equally likely to carry the A allele or the a allele, but never both or neither. The fact that homologous chromosomes, and thus homologous genes, segregate to opposite poles at the first meiotic division explains this principle of inheritance. See also: Allele
Mendel's law of independent assortment states that the segregation of two different gene pairs occurs at random with respect to each other. Thus, for an individual of the genotype AaBb, the gametes AB, Ab, aB, and ab will be formed with equal frequency. This result can be easily understood if the A and B genes lie on different chromosomes (Fig. 2). Because the chromosome pair bearing the A gene orients independently of the homolog pair bearing the B gene, AB ↔ ab segregations are as likely as Ab ↔ aB segregations. Cases where independent assortment does not occur (an effect called linkage) can be understood as resulting from situations where the two gene pairs lie at different positions on the same pair of homologous chromosomes. See also: Gene; Linkage (genetics); Mendelism
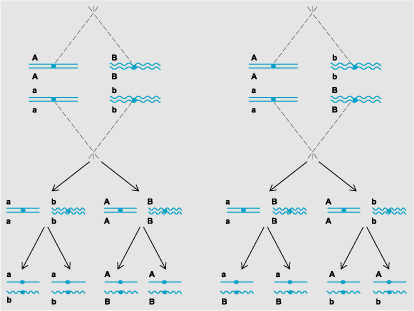
Meiotic divisions
The two meiotic divisions (meiosis I and II) may be divided into a number of distinct stages. Meiotic prophase refers to the period after the last cycle of DNA replication; during prophase, homologous chromosomes pair and recombine. The end of prophase is signaled by the breakdown of the nuclear envelope and the association of the paired chromosomes with the meiotic spindle. The spindle is made up of microtubules that, with associated motor proteins, mediate chromosome movement. In some cases (for example, human sperm formation), the spindle is already formed at the point of nuclear envelope breakdown, and the chromosomes then attach to it. In other systems (for example, human female meiosis), the chromosomes themselves organize the spindle.
Metaphase I is the period before the first division when pairs of interlocked homologous chromosomes, called bivalents, line up on the middle of the meiotic spindle. The chromosomes are primarily (but not exclusively) attached to the spindle by their centromeres; the centromere of one homolog is attached to spindle fibers emanating from one pole, and the centromere of its partner is attached to spindle fibers from the other pole (Fig. 3). The bivalents are physically held together by structures referred to as chiasmata, which are the result of meiotic recombination events. In most meiotic systems, meiosis will not continue until all of the homolog pairs are properly oriented at the middle of the spindle—the metaphase plate. It is important to remember that the orientation of each pair of homologs on the spindle occurs in a random fashion, such that the paternally derived homolog of one bivalent may point toward one pole of the spindle, whereas the maternally derived homolog in the adjacent bivalent is oriented toward the same pole.
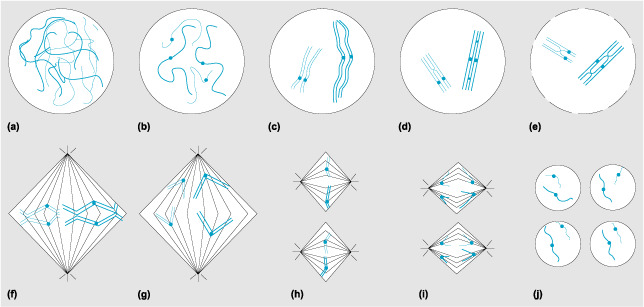
Anaphase I refers to the point at which homologous chromosome pairs separate and move to opposite poles. This is accomplished by the release of chiasmata. Although the sister chromatids remain attached around their centromeres, they release each other along the arms of the chromosome, allowing chiasmata to be resolved. Depending on the organism, there may or may not be a true telophase, or a time in which nuclei reform. In most organisms, the first cell division occurs after the completion of anaphase I.
Following the completion of the first meiotic division, the chromosomes align themselves on a new pair of spindles, with their sister chromatids oriented toward opposite poles. The stage at which each chromosome is so aligned is referred to as metaphase II. In some (but not all) organisms, metaphase II is preceded by a brief prophase II. DNA replication does not occur during prophase II; each chromosome still consists of the two sister chromatids. In addition, there are no opportunities for pairing or recombination at this stage due to the prior separation of homologs at anaphase I.
The start of anaphase II is signaled by the separation of sister centromeres and the movement of the two sister chromatids to opposite poles. At telophase II, the sisters have reached opposite poles and the nuclei begin to reform. The second cell division usually occurs at this time. Thus, at the end of the second meiotic division, there will be four daughter cells, with each having a single copy of each chromosome.
Details of meiotic prophase
Pairing and recombination occur during the first meiotic prophase, which is divided into a number of stages: leptotene, zygotene, pachytene, diplotene, and diakinesis (Fig. 3). Homolog recognition, alignment, and synapsis (pairing) occur during leptotene and zygotene. In the leptotene stage (an initial phase of chromosome individualization), initial homolog alignments are made. By zygotene, homologous chromosomes have become associated at various points along their length. These associations facilitate a more intimate pairing that results in the homologous chromosomes lying abreast of a tracklike structure called the synaptonemal complex. The beginning of pachytene is signaled by the completion of a continuous synaptonemal complex running the full length of each bivalent. During diplotene, the attractive forces that mediated homologous pairing disappear, and the homologs begin to repel each other. However, homologs virtually always recombine, and those recombination events can be seen as chiasmata that tether the homologs together. In some organisms, those rare chromosome pairs that have failed to undergo recombination will fly apart prematurely from each other at this stage. The final stage in meiotic prophase is diakinesis, during which the homologs shorten and condense in preparation for nuclear division.
Recombination
Meiotic recombination (Fig. 4) involves the physical interchange of DNA molecules between the two homologous chromosomes, thus allowing the creation of new combinations of alleles for genes located on that pair of chromosomes. Mechanistically, recombination occurs between homologous chromosomes and involves the precise breakage and rejoining of two nonsister chromatids. The result is the formation of two recombinant chromatids, with each carrying information from both of the original homologs. See also: Recombination (genetics)
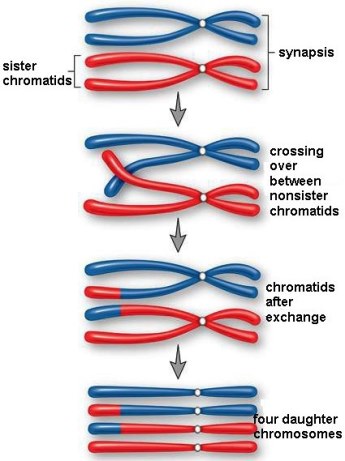
The actual mechanism of recombination involves a complex series of cutting and rejoining of homologous DNA. The number and position of recombination events are very precisely controlled. Exchange occurs only in the gene-rich euchromatin that makes up most of the chromosome arms; it never occurs in the heterochromatin that surrounds the centromeres. Moreover, as a result of a process known as interference, the occurrence of one exchange in a given chromosomal region greatly decreases the probability of a second exchange in that region.
Sex differences in meiosis
In human male meiosis, all four daughter cells of meiosis will go through a complicated cellular differentiation process called spermatogenesis to become mature functional sperm. In contrast, oogenesis in the human female results in only one of the four products of meiosis becoming an egg. The other three products donate their cytoplasm to the chosen oocyte, and then die. The oocyte then completes the cellular differentiation process to become a mature egg. See also: Oogenesis; Spermatogenesis
In human females, meiosis begins during fetal development. All of the oocytes (eggs) that a human female will possess in her lifetime are produced during fetal development, but these oocytes are arrested at the end of pachytene. Thus, all of the meiotic recombination that a human female will ever do is completed before she is born. These arrested oocytes remain quiescent until the female enters puberty. At that point, a few oocytes are allowed to begin the maturation process during each menstrual cycle; usually only a single oocyte is ovulated per cycle. The ovulated egg completes anaphase I and then proceeds through meiosis until it arrests again at metaphase II. The completion of the second meiotic division is triggered only by fertilization.
Male meiosis begins at puberty and continues uninterrrupted throughout the life of the male. The stem cells that will produce male meiotic cells continue to divide throughout the male's life, constantly producing new populations of spermatocytes. Moreover, once meiosis is initiated in human spermatocytes, it usually proceeds without interruption to produce four daughter cells; then, all of these differentiate to become mature spermatids.
Errors of meiosis
The failure of two chromosomes to segregate properly is called nondisjunction. Nondisjunction occurs either because two homologs failed to pair and/or recombine or because of a failure of the cell to properly move the segregating chromosomes on the meiotic spindle. The result of nondisjunction is the production of gametes that are aneuploid, that is, carrying the wrong number of chromosomes. When such a gamete is involved in a fertilization event, the resulting zygote is also aneuploid. Those cases where the embryo carries an extra copy of a given chromosome are said to be trisomic, whereas those that carry only one copy are said to be monosomic for that chromosome. Most aneuploid zygotes are not viable and result in early spontaneous abortion. There are no viable monosomies for the human autosomes (non-sex chromosomes). However, a few types of trisomic zygotes are capable of survival; these are trisomies for the sex chromosomes (XXX, XXY, and XYY), trisomy 21 (Down syndrome), trisomy 18, and trisomy 13. See also: Chromosome aberration; Crossing-over (genetics); Down syndrome
Meiosis versus mitosis
The fundamental difference between meiosis and mitosis is that sister chromatids do not separate at the first meiotic division; rather, homologous chromosomes separate from each other with their sister chromatids still attached to each other. Although some chromosome pairing does occur in mitotic cells in some organisms, intimate synapsis along the entire length of chromosomes is usually restricted to meiotic cells. Recombination is frequent in most meiotic cells; however, it occurs only rarely in mitotic cells, usually as part of DNA repair events. Most critically, DNA synthesis occurs only once within the two meiotic divisions. In contrast, there is a complete replication before every mitotic division. This allows mitosis to produce two genetically identical daughter cells, whereas meiosis produces four daughter cells, with each having only one-half of the number of chromosomes present prior to meiosis. See also: DNA repair; Mitosis