Key Concepts
The making of a compound from available starting materials by one or more steps. Organic synthesis plays an important role in chemistry, biochemistry, medicine, agriculture, molecular biology, physics, materials science, electronics, and engineering by allowing for the creation of specific molecules for scientific and technological investigations. See also: Organic chemistry
Synthetic strategy
The heart of organic synthesis is designing synthetic routes to a molecule. The simplest synthesis of a molecule is one in which the target molecule can be obtained by using a readily available starting material for a single reaction that converts it to the desired target molecule. However, in most cases the synthesis is not that straightforward. To convert a chosen starting material to the target molecule, numerous steps that add, change, or remove functional groups, and steps that build up the carbon atom framework of the target molecule may need to be done (Fig. 1).
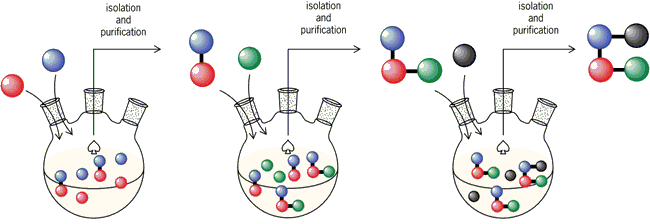
Retrosynthetic analysis
A systematic approach for designing a synthetic route to a molecule is to subject the target molecule to an intellectual exercise called a retrosynthetic analysis. This involves an assessment of each functional group in the target molecule and the overall carbon atom framework in it; a determination of what known reactions from each of those functional groups or that build up the necessary carbon framework as a product; and a determination of what starting materials (synthetic precursors or synthetic intermediates) for each such reaction are required. The resulting starting materials are then subjected to the same retrosynthetic analysis, thus working backward from the target molecule until starting materials that are commercially available (or available by synthesis following an already published procedure) are derived. See also: Reactive intermediates
The retrosynthetic analysis of a target molecule usually results in more than one possible synthetic route. It is therefore necessary to critically assess each derived route in order to choose the single route that is most feasible (most likely to proceed as written, with as few unwanted side reactions as possible) and most economical (involving the fewest steps and using the least expensive starting materials). The safety of each possible synthetic route (the toxicity and reactivity hazards associated with the reactions involved) is also considered when assessing alternative synthetic routes to a molecule.
A retrosynthetic analysis can be done by using computer programs to derive as comprehensive a list of possible synthetic routes to a target molecule as possible. One important aspect of synthetic planning is that it depends upon a knowledge (by a chemist or computer program) of known synthetic transformations, reactions that build up carbon skeletons or introduce functional groups or interconvert functional groups.
Stereoselectivity
Selectivity is an important consideration in the determination of a synthetic route to a target molecule. Stereoselectivity refers to the selectivity of a reaction for forming one stereoisomer of a product in preference to another stereoisomer. Stereoselectivity cannot be achieved for all organic reactions; the nature of the mechanism of some reactions may not allow for the formation of one configuration of a chiral (stereogenic) carbon center or one particular geometry (cis versus trans) for a double bond or ring. When stereoselectivity can be achieved in a reaction, it requires that the reaction proceed via a geometrically defined transition state and that one or both of the reactants possess a particular geometrical shape during the reaction. In many reactions, if one or both of the reactants is chiral, the absolute configuration of the newly formed stereogenic carbon center can be selectedr. Nucleophilic substitution is an example of a reaction that can proceed stereoselectively when the starting material is chiral. Pericyclic reactions also proceed stereoselectively, because they involve transition states that have well-defined geometries. The achievement of stereoselectivity is an important aspect of organic synthesis, because usually a single stereoisomer of a target molecule is the desired goal of a synthesis. Sometimes the target molecule contains a chiral (stereogenic) carbon center; that is, it can exist as either of two possible enantiomers. The possible synthetic routes to the target molecule may not be selective for forming a single enantiomer of the target molecule; each would form a racemic mixture. In some cases, such nonstereoselective synthetic routes to a molecule are acceptable. However, if a synthesis of a single stereoisomer of a target molecule is required, the stereoselectivity of the reactions derived during the retrosynthetic analysis would need to be considered. The development of stereoselective reactions is an active area of research in organic synthesis. See also: Asymmetric synthesis; Organic reaction mechanism; Pericyclic reaction; Racemization; Stereochemistry
Chemoselectivity
This term refers to the ability of a reagent to react selectively with one functional group in the presence of another similar functional group. An example of a chemoselective reagent is a reducing agent that can reduce an aldehyde and not a ketone. In cases where chemoselectivity cannot be achieved, the functional group that should be prevented from participating in the reaction can be protected by converting it to a derivative that is unreactive to the reagent involved. The usual strategy employed to allow for such selective differentiation of the same or similar groups is to convert each group to a masked (protected) form which is not reactive but which can be unmasked (deprotected) to yield the group when necessary.
Synthetic reactions
A large variety of organic reactions that can be used in syntheses are known. They can be categorized according to whether they feature a functional group interconversion or a carbon-carbon bond formation.
Functional group interconversions (Fig. 2) are reactions that change one functional group into another functional group. A functional group is a nonhydrogen, non-all-singly-bonded carbon atom, or group of atoms. Included in functional group interconversions are nucleophilic substitution reactions, electrophilic additions, oxidations, and reductions. See also: Computational chemistry; Electrophilic and nucleophilic reagents; Oxidation-reduction; Oxidizing agent; Substitution reaction
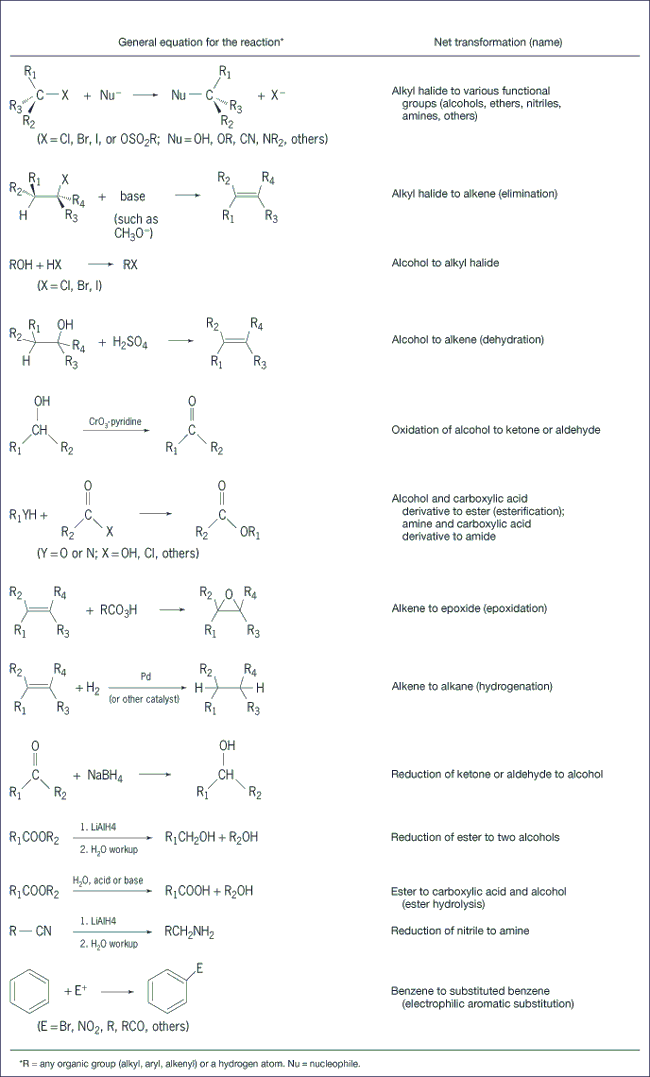
Carbon-carbon bond-forming reactions (Fig. 3) feature the formation of a single bond or double bond between two carbon atoms. This is a particularly important class of reactions, as the basic strategy of synthesis—to assemble the target molecule from simpler, hence usually smaller, starting materials—implies that most complex molecules must be synthesized by a process that builds up the carbon skeleton of the target by using one or more carbon-carbon bond-forming reactions. See also: Chemical bonding; Diels-Alder reaction; Grignard reaction; Rearrangement reaction
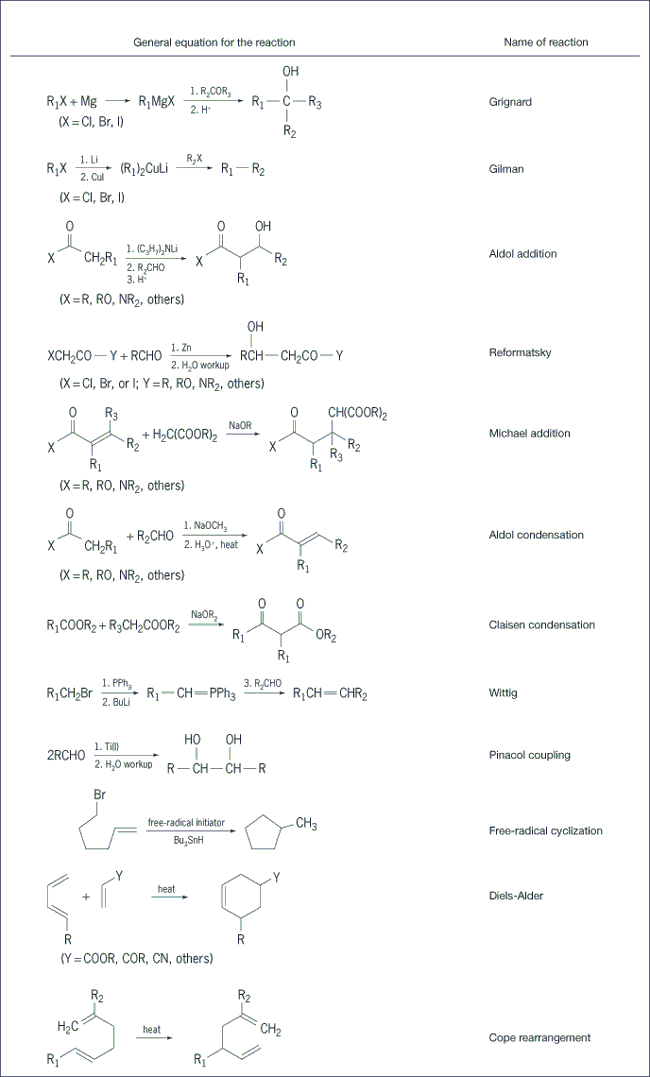
Total synthesis
Total synthesis is the laboratory construction of a complex molecule, often a natural product, through a series of reactions using relatively simple and commercially available molecules as starting materials. In contrast, semisynthesis starts with larger molecules, also often from naturally occurring sources, with fewer reactions needed to reach the final product.
In its early days, total synthesis was used as a means of verifying chemical structures. As analytical chemistry progressed and chemical structures could be determined by instrumental analysis, researchers moved on to synthesizing complex molecules of biological, medicinal, or material importance. Today the emphasis in total synthesis is on what is sometimes called “ideal” synthesis: the efficient production of complex molecular structures in as few steps as possible. Step and atom economy are green chemistry; they improve synthetic efficiency by increasing yield and reducing waste and time. However, new reactions are needed to produce large quantities of molecules in a practical and environmentally friendly way. See also: Analytical chemistry; Atom economy; Bioorganic chemistry; Green chemistry
The basic steps of total synthesis are isolation, characterization, and a synthetic strategy. First a compound of interest (target molecule) must be isolated and characterized to determine its structure and functional properties (such as its pharmacological importance). To develop a synthetic strategy, investigators do a retrospective (retrosynthetic) analysis, working backward from the target molecule to identify a series of reactions that would produce it from the available starting materials. In constructing a retrosynthetic strategy, the analysis must consider the atoms, bonds, functional groups, conformational properties, and stereocenters of the target molecule. See also: Conformational analysis; Pharmacology
A classic example of a molecule derived from total synthesis is the monocyclic beta-lactam antibiotic aztreonam (Fig. 4), a structurally modified compound originally isolated from Chromobacterium violaceum, a gram-negative rod-shaped bacterium. Aztreonam is produced synthetically and is used for treating infections with gram-negative bacteria. See also: Antibiotic; Bacteria
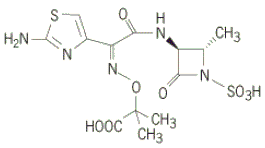