Key Concepts
An elementary particle that is the force carrier of the electromagnetic force and a quantum of electromagnetic radiation, also known as light. Photons are the most common elementary particle in the universe described by the standard model of particle physics. Having zero rest mass, photons always move at their namesake speed of light, which in a vacuum is 299,792,458 m/s, irrespective of their energy (Fig. 1). See also: Electromagnetic radiation; Electromagnetism; Elementary particle; Energy; Light; Mass; Standard model; Universe
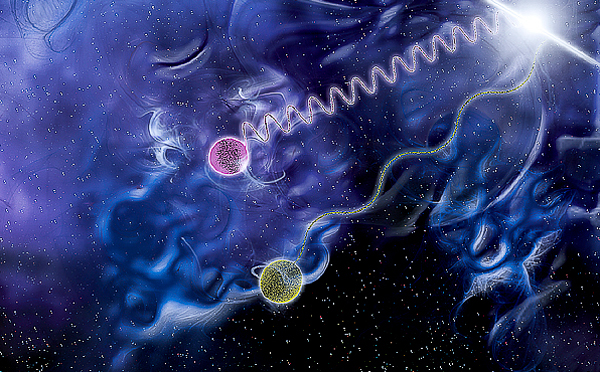
As conveyors of the electromagnetic force between charged particles such as electrons and protons, photons are, by human reckoning, conspicuously involved in the interactions of matter as a type of energy called light. Our eyes and brain perceive photons that possess a narrow range of frequencies or wavelengths within the electromagnetic spectrum as visible light. We can "feel" or be otherwise physiologically affected by photons of certain higher or lower energies. Instruments, particularly for astronomy, are used to detect the full range of the electromagnetic spectrum, from the high-energy gamma rays down to low-energy radio waves (Fig. 2). See also: Astronomy; Electric charge; Electron; Electroweak interaction; Frequency (wave motion); Frequency measurement; Fundamental interactions; Infrared radiation (biology); Ultraviolet radiation (biology); Vision; Wavelength
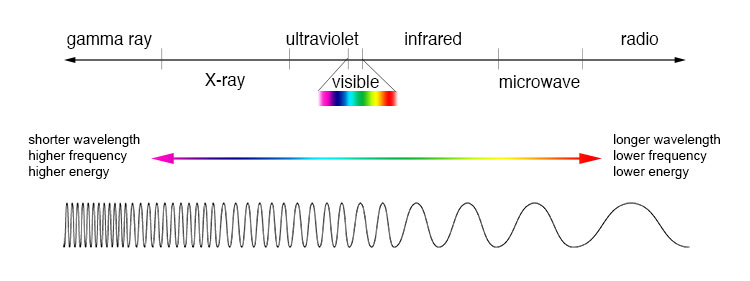
According to classical electromagnetic theory, an electromagnetic wave can transfer arbitrarily small amounts of energy to matter. According to the quantum theory of radiation, however, the energy is transferred in discrete amounts. The energy of a photon is the product of Planck's constant and the frequency of the electromagnetic field. In addition to energy, the photon possesses momentum and also possesses angular momentum corresponding to a spin of unity. The interaction of radiation with matter involves the absorption, scattering, and emission of photons. Consequently, the energy interchange is inherently quantized. See also: Absorption; Absorption of electromagnetic radiation; Angular momentum; Energy; Momentum; Spin (quantum mechanics)
Wave-particle duality
As with other elementary particles, photons are best described as having a wave-particle duality within the framework of quantum mechanics. Photons can constructively and destructively interfere with each other as waves, yet also can be measured and produced as discrete particles during experiments and in various technologies. See also: Electromagnetic wave; Electromagnetic wave transmission; Interference of waves; Quantum mechanics; Wave-particle duality
Particlelike behavior
For many purposes, the photon behaves like a particle of zero rest mass moving at the speed of light. Unlike spin-1 particles of finite mass in the standard model, the photon has only two, rather than three, polarization states. This corresponds to the transverse nature of a classical electromagnetic wave in free space. The particlelike nature of the photon is vividly exhibited by the photoelectric effect, predicted by German-born U.S. theoretical physicist Albert Einstein, in which light is absorbed in a metal, causing electrons to be ejected. An electron absorbs a photon, gaining its energy. In leaving the metal, it loses energy because of interactions with the surface; the energy loss equals the product of the so-called work function of the surface and the charge of the electron. The final kinetic energy of the electron therefore equals the energy of the incident photon minus this energy loss. See also: Metal; Photoemission; Polarization of waves
A second demonstration of the particlelike behavior of photons is provided by the scattering of an x-ray photon from an electron bound in an atom. The electron recoils because of the momentum of the photon, thereby gaining energy. As a result, the frequency, and hence the wavelength of the scattered x-ray, is altered. If the x-ray is scattered through a certain angle, the wavelength is shifted by an amount determined by this scattering angle and the mass of an electron, according to the laws of conservation of energy and momentum. See also: Compton effect; X-rays
Quantum theory
From a more fundamental view, the photon is the quantum of excitation of a single mode of a radiation field. The dynamical equations for the electric and magnetic energy in such a field are identical to those of a harmonic oscillator. According to quantum theory, the allowed energies of a harmonic oscillator are given by E = ( j + ½)h f, where h is Planck's constant, f is the frequency of the oscillator, and the quantum number j = 0, 1, 2, . . ., describes the state of excitation of the oscillator. This quantum relation was first postulated by M. Planck for the material oscillators in the walls of a thermal enclosure in order to obtain the correct form for the density of radiation in a thermal field, but it was quickly applied by Einstein to describe the state of the radiation field itself. In this picture, j describes the number of photons in the field. See also: Harmonic oscillator
Many of the processes by which radiation interacts with matter can actually be described without employing a quantized description of the radiation field or the concept of photons, that is, by treating the radiation field classically. However, the fundamental process of spontaneous emission, in which an excited atom or molecule emits a photon and makes a transition to its ground state, can be described only on the basis of a quantized field. Also, many statistical properties of the radiation field, for instance correlations between photons that are emitted simultaneously in a nonlinear process, are inexplicable in terms of classical fields but have a natural explanation in the quantum theory of radiation and the concept of photons. See also: Nonrelativistic quantum theory; Quantum electrodynamics; Quantum field theory; Quantum mechanics; Relativistic electrodynamics; Relativistic quantum theory
Technological applications
The rich theoretical understanding of light as a classical phenomenon—as well as more granularly and quantum mechanically as the photon—and how to manipulate generation, reception, and other properties is behind many revolutionary technologies, such as radio communications, lasers, and optical fibers, to name a few. An important new frontier is the controlling of individual photons for use in quantum computers and for quantum cryptography. See also: Fiber-optic circuit; Laser; Optical communications; Optical fibers; Quantum communications; Quantum computation