Key Concepts
The reception of electromagnetic radiation by particles with electric charge, resulting in transference of the radiation's energy and momentum. Electromagnetic radiation is also referred to as electromagnetic waves, photons, and light. Photons are the elementary particles that carry electromagnetic energy, manifesting as light across a spectrum of energies, or wavelengths. Light can be absorbed by any particle that carries electric charge, such as an electron. The light that is absorbed is destroyed, in the sense that its energy and momentum are transferred to the charged particle, with individual photons of light ceasing to exist. The absorbed energy can increase the charged particle’s kinetic energy, potential energy, or both, leading to effects such as heating (Fig. 1) and atomic transitions. The absorbed momentum can change the particle’s motion, leading to effects such as ionization and radiation pressure. See also: Electric charge; Electromagnetic radiation; Electromagnetism; Electron; Elementary particle; Energy; Heat; Ionization; Light; Momentum; Motion; Photon
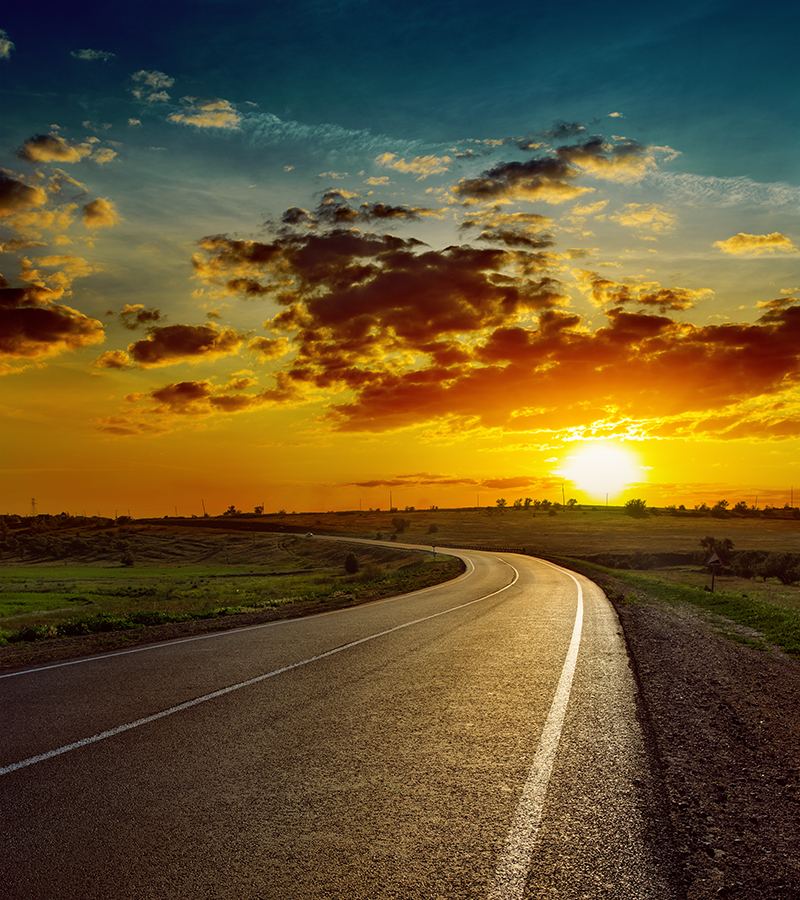
The visible appearance of objects
The visible appearance of an object is determined by how well it absorbs, transmits, and reflects the various colors of light. Colors of visible light correspond to particular photon wavelengths. See also: Color; Reflection of electromagnetic radiation
- A black object such as coal absorbs all colors.
- A white object such as snow reflects all colors diffusely.
- A silvery object such as a mirror reflects all colors specularly.
- An opaque colored object such as a rose reflects some colors and absorbs the other colors.
- A transparent object such as a window pane transmits all colors.
- A semitransparent object or translucent object such as stained glass transmits some colors and absorbs the other colors.
- A transparent thin film such as a soap bubble transmits some colors and reflects the other colors.
Quantum effects
All electromagnetic phenomena, including absorption, are governed by the quantum version of Maxwell’s equations, which are known collectively as quantum electrodynamics (QED). In 1861, Scottish physicist James Clerk Maxwell systematically organized all the laws of electricity and magnetism into one complete set. Throughout the twentieth century, a wide array of scientists quantized Maxwell’s equations and established QED. These equations state that electromagnetic radiation consists of quantized, traveling fluctuations in the electromagnetic field. Electromagnetic radiation exists across a wide range of frequencies and can be organized on a frequency spectrum as follows: radio waves, infrared waves, visible light, ultraviolet rays, x-rays, and gamma rays. See also: Electricity; Magnetism; Maxwell's equations; Quantum electrodynamics
Electromagnetic radiation is composed of indivisible quantum packets called photons that each carry a fixed amount of energy and momentum. Whenever charged particles absorb electromagnetic radiation, the radiation must be absorbed as a whole number of photons. For typical situations, too many photons are present to allow the quantized nature of absorption to be noticeable. The counting of individual photon absorption events requires sensitive devices and low-intensity light. Photons can be absorbed by free particles or by particles bound in atoms or molecules. See also: Atom; Molecule
Types of absorption
Absorption by free charged particles
When electromagnetic radiation is absorbed by a free charged particle, it gives the particle additional kinetic energy. This causes the particle to change its state of motion, which can manifest as heat or an electric current. See also: Electric current
Plasma absorption
Plasma absorption, also known as inverse bremsstrahlung, involves the absorption of electromagnetic radiation by the free electrons and ions of a plasma. In this process, the energy that is absorbed becomes thermal energy and the plasma heats up. An example of this occurs in nuclear fusion reactors. See also: Bremsstrahlung; Ion; Nuclear fusion; Plasma (physics)
Antenna absorption
When electromagnetic radiation is absorbed by the conduction electrons that are free to move in an antenna, it pushes the electrons along the antenna, causing a measurable electric current. This is known as antenna absorption or radio reception. The absorbed energy and information can then be detected and analyzed by an electric circuit. Examples of this include the antennas in cell phones, radar receivers, and satellite dishes. See also: Antenna (electromagnetism); Mobile communications
Absorption by bound charged particles that become free
When electromagnetic radiation is absorbed by a bound charged particle, it can give the particle enough energy to set it free. An electron can be freed from an atom, a molecule, or from the energy band of a solid.
Ionization
Ionization involves the freeing of an electron that was bound in an atom or small molecule. The atom or molecule that is left behind loses an electron and therefore becomes a positive ion. Often, the free electron and ion react aggressively with neighboring atoms. For this reason, ionization in living tissue can lead to mutation, cancer, or radiation sickness; ionization in materials can damage them. Of the various frequencies on the electromagnetic spectrum, only extreme ultraviolet rays, x-rays, and gamma rays have enough energy per photon to be ionizing. Other mechanisms besides photon absorption can cause ionization. Accordingly, the ionization that results from photon absorption is often called photoionization. See also: Cancer; Mutation; Radiation injury to plants and animals
The photoelectric effect
Electromagnetic radiation can also free an electron that is bound in a solid or a large molecule. Such electrons are more loosely bound than electrons that are bound inside atoms or small molecules, and therefore can be set free by non-ionizing radiation such as visible light. This process is known as the photoelectric effect. Note that the term photoelectric effect is sometimes used broadly to refer to any type of photon absorption. The photoelectric effect is used in devices such as night-vision goggles (Fig. 2) and photomultiplier tubes. It is also the key step in photosynthesis. See also: Photoemission; Photomultiplier; Photosynthesis
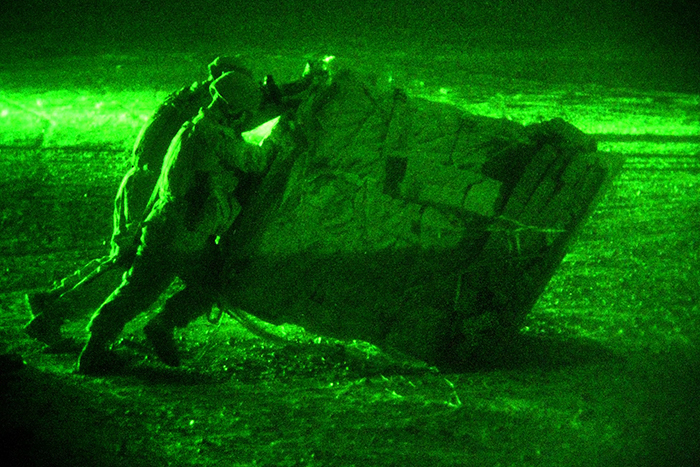
Absorption by bound charged particles that remain bound
When non-ionizing radiation such as visible light is absorbed by an electron bound in an atom or small molecule, the radiation cannot give the electron enough energy to set it free. Instead, the electron is excited to a higher-energy atomic or molecular state. See also: Excitation; Excitation potential
Dissipative absorption
Dissipative absorption is the conversion of the energy of the absorbed electromagnetic radiation to thermal energy, which heats up the object. This is the most common type of absorption in everyday life. Light that is absorbed by everyday objects such as tables and chairs is continuously being converted to thermal energy.
Energy-band transition
In solids, an electron that is not bound deep inside an atom or molecule exists in a delocalized state within an energy band. In many situations, the energy from an absorbed photon may give such an electron enough energy to transition to another energy band. In metals and semiconductors, energy-band transitions can be easily controlled and measured. This is the operating principle behind solar panels, photographic film, and digital cameras. See also: Band theory of solids; Conduction band; Digital photography; Metal; Semiconductor; Solar energy
Fluorescence
In certain materials, an electron excited to a higher-energy atomic or molecular state by photon absorption must return to its original state through an intermediate state. As the electron transitions back, it emits photons. Because the electron’s return path involves smaller energy steps, the photons that it emits have lower energy and thus lower frequency than the original photons that it absorbed. This process is known as fluorescence. The most common type of fluorescence occurs when a material converts ultraviolet light to visible light (Fig. 3), making the material seem unnaturally bright. Examples include fluorescent paper and safety vests. See also: Fluorescence
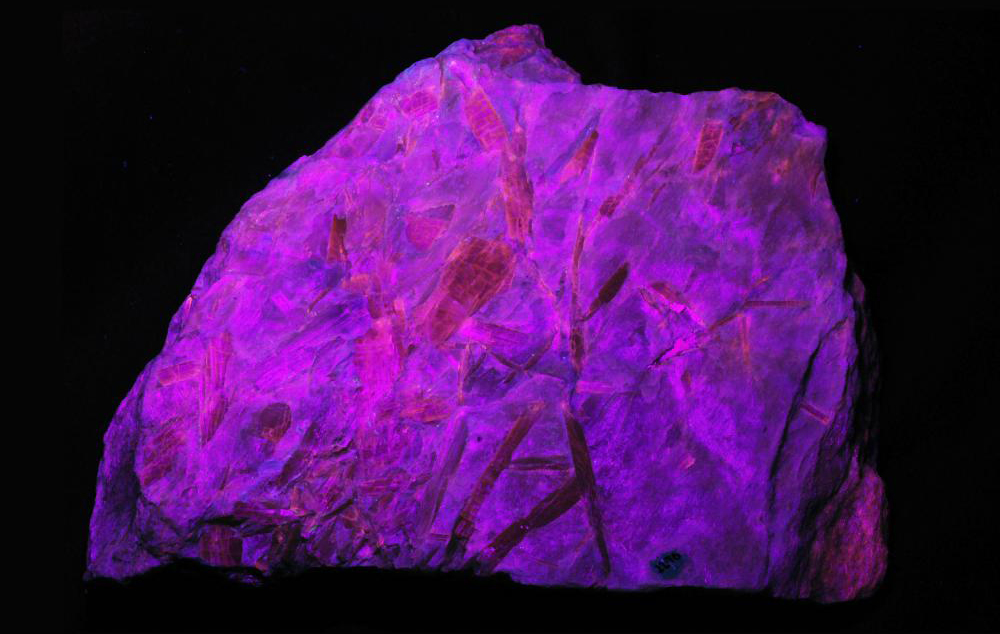
Phosphorescence
Phosphorescence is conceptually the same as fluorescence in that it involves the absorption of photons and the subsequent emission of lower-frequency photons. The difference is that phosphorescence involves an electron transition that takes a relatively long time to occur. As a result, the material can continue to emit light for many hours after the original light source has been removed. In everyday language, phosphorescent objects are known as glow-in-the-dark objects. Examples include glow-in-the-dark stickers and paint. See also: Phosphorescence
Vision
Vision is the absorption of light by biological organisms in such a way that information can be extracted. Photoreceptors in the eye are structured so that the excitation of an electron by photon absorption leads to a cascade of chemical events. These events ultimately send electrical signals to the brain, allowing the perception of images. See also: Eye (vertebrate); Vision
Particle–antiparticle creation
When two gamma-ray photons meet under the right conditions, the photons can mutually destroy each other and create a particle-antiparticle pair. The most common result is the creation of an electron and a positron. This process is very rare. See also: Antimatter
Beam attenuation and the Beer-Lambert law
For semitransparent materials, electromagnetic radiation is able to penetrate through the material but is partially absorbed as it travels. The radiation becomes steadily weaker as it advances through the material because its photons are being steadily destroyed. This effect is known as beam attenuation. Because beam attenuation is caused by photon absorption, the rate of attenuation depends on the amount of absorptive atoms and molecules that are present. This principle is summarized in the Beer-Lambert law, established by German scientist August Beer in 1852. It states that for a uniform material containing one type of absorbing atom or molecule, T = e -σnl . In this equation, T is the percent of radiant electromagnetic energy transmitted per second, σ is the attenuation cross section of each atom or molecule, n is the number density of absorbing atoms or molecules, and l is the length of the sample in the direction the radiation is traveling. An example of beam attenuation is the fact that the ambient light level in the deep ocean decreases with depth. See also: Attenuation