Key Concepts
The interaction of the behavior and ecology of hosts with the biology of pathogens as relating to the impact of diseases on populations. Although human diseases have been studied from an ecological perspective for centuries, diseases in plant and animal populations have received much less attention. The reason that ecologists have generally overlooked diseases is that diseases tend to be "invisible" compared to predators or competitors. The toll that diseases can take on all organisms (even bacteria are attacked by diseases) is enormous, and the ecology of diseases is one of the most vigorous areas of research in modern biology (Fig. 1). In addition, the role of natural ecosystems in diseases and the possibility that human activities can profoundly alter the ecology of diseases are very significant. See also: Disease; Ecology; Ecosystem; Pathogen; Pathology; Physiological ecology (animal); Physiological ecology (plant); Population ecology
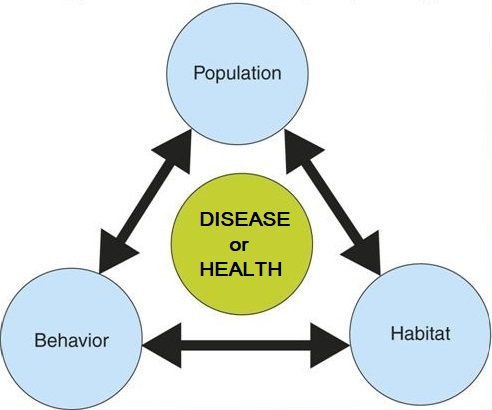
Threshold theorem
For a disease to spread, it must be successfully transmitted to a new host before its current host dies or recovers. This observation lies at the core of the most important idea in epidemiology: the threshold theorem. The threshold theorem states the following: if the density of susceptible hosts is below some critical value, then the transmission of a disease, on average, will not occur rapidly enough to cause the number of infected individuals to increase. In other words, the reproductive rate of a disease must be greater than 1 for there to be an epidemic, with the reproductive rate being defined as the average number of new infections created per infected individual. See also: Ecological modeling; Epidemiology
Human immunization programs are based on applying the threshold theorem of epidemiology to public health; specifically, if enough individuals in a population can be vaccinated, then the density of susceptible individuals will be sufficiently lowered so that epidemics are prevented. Certain diseases, such as smallpox (Fig. 2), have been eradicated because vaccination rates were high enough worldwide (70–80% immunization rates) to preclude the possibility of a smallpox outbreak. More generally, because diseases spread rapidly when densities of hosts are high, and less effectively (or not at all) when host densities are low, diseases can profoundly influence the population dynamics of their hosts—either by causing fluctuations in host density or by regulating host densities to low levels in conjunction with other factors. Even human populations seem to have been dramatically influenced by diseases. For example, between the fifth and eleventh centuries, European populations were ravaged by cycles of the plague. The initial plague epidemics killed 25–40% of the urban populations in Europe. Later cycles of plague were less deadly because the populations had evolved resistance, and individuals had acquired immunity. See also: Immunity; Plague; Smallpox; Vaccination
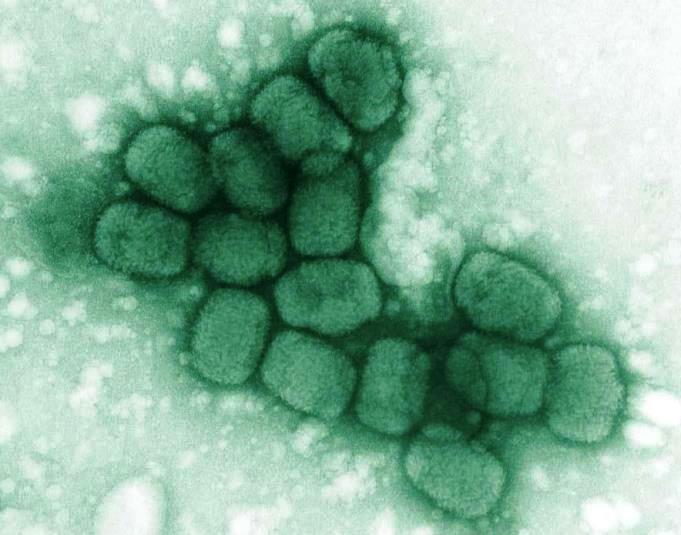
Diseases have profoundly altered the course of human history. For example, the conquest of the Aztecs by a few hundred Spanish soldiers was due primarily to the fact that the Spaniards brought new diseases to the Western Hemisphere that decimated the Aztec populations. In particular, the population of Mexico fell from about 20 million to 3 million between 1518 and 1568. The deaths were from successive epidemics of smallpox, measles, and typhus (a rickettsial disease), which were diseases that had never been seen previously in the New World, but to which the Europeans had built up a resistance (after centuries of exposure). New diseases continue to have a major impact on humans (for example, the emergence in 2019 of SARS-CoV-19, a novel coronavirus that causes the disease COVID-19). See also: Aztecs; Coronavirus; Epidemic; Measles; Rickettsioses; The COVID-19 pandemic
In general, the rate of reproduction for diseases is proportional to their transmissibility and to the length of time that an individual is infectious. For this reason, extremely deadly diseases that kill their hosts too rapidly may require extremely high densities of hosts before they can spread. In addition, all diseases do not behave as simply as hypothesized by the threshold theorem; the most notable exceptions are sexually transmitted diseases. Because organisms actively seek reproduction, the rate at which a sexually transmitted disease is passed among hosts is generally much less dependent on host density. For this reason, sexually transmitted diseases are especially hard to control. See also: Sexually transmitted diseases
Population effects
Cycles in many animal populations are thought to be driven by diseases. For example, the population fluctuations of larch bud moths in Europe are hypothesized to be driven by a virus that infects and kills the caterpillars of this species. Similarly, population fluctuations of red grouse in northern England are also thought to be driven by disease; in this case, parasitic nematodes are the infectious agents. The interaction between parasitic worms and red grouse is subtle. In particular, the presence of a worm infection does not necessarily mean that a grouse is harmed; instead, it is only when grouse are laden with heavy worm burdens that effects are seen, and those effects take the form of reduced breeding success or higher mortality during the winter. This example highlights a common feature of diseases: their effects may be obvious only when their hosts are assaulted by other stresses as well (such as harsh winters and starvation). The most severe human plagues in the Middle Ages were associated with times of famine, presumably because malnourished victims were less able to withstand the plague. See also: Nemata (Nematoda); Parasitology; Virus
In addition, it is widely appreciated that plants, including crop plants, are attacked by a tremendous diversity of diseases. However, the toll of these diseases seems to be reduced (in comparison to the toll of diseases in animals) because natural plant populations are so genetically variable that it is unlikely that any given pathogen strain can sweep through and kill all of the plants; that is, there are always some resistant genotypes. If severe enough, though, these diseases are capable of ruining an entire year's production; for example, a corn leaf blight (Fig. 3) destroyed approximately one-third of the United States corn crop in 1970. Furthermore, when agronomists have bred plants for uniformity, they have often depleted genetic diversity and created a situation in which a plant pathogen that evolves to attack the crop encounters plants with no resistance (all the plants are the same) and sweeps through the fields with drastic consequences. For example, when the aforementioned leaf blight devastated the corn crop in the United States, 70% of that crop shared genetically identical cytoplasm, and the genetic uniformity of the host exacerbated the severity of the epidemic. In fact, hundreds of agricultural experiments have been performed in which the severity of plant diseases is contrasted between fields planted with a mixture of varieties and fields planted with only one variety. The results reveal that, on average, genetic variety reduces disease incidence by 50% compared to genetically homogeneous (only one variety) plantings. This is one reason why biodiversity and genetic diversity are viewed as a natural resource; such diversity is likely to harbor resistance traits, which can be bred (or transferred by genetic engineering) into food crops. See also: Genetic engineering; Genetically engineered plants; Genetically modified crops; Genetics; Plant breeding; Plant pathology
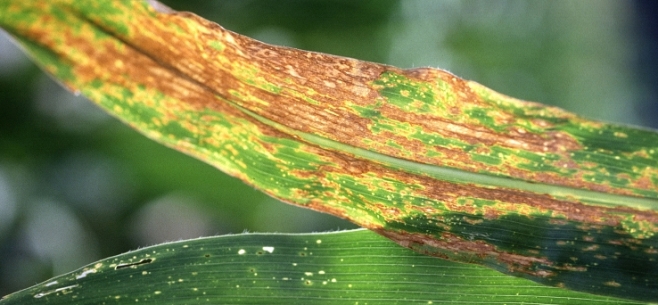
Disease emergence
Humans are dramatically altering habitats and ecosystems. Sometimes, these changes can influence disease interactions in surprising ways. Lyme disease provides a good example of the interplay of human habitat modifications and diseases. Lyme disease involves a spirochete bacterium transmitted to humans by ticks. However, humans are not the normal hosts for this disease; instead, both the ticks and the bacterium are maintained primarily on deer and mice populations. Human activities influence both deer and mice populations, and in turn tick populations, affecting potential exposure of humans to the disease. The pathways for this influence are numerous and hard to predict. Moreover, one well-documented human effect has arisen from the control of natural predators of deer and the clearing of forest habitat; both changes have favored the buildup of large deer populations. As deer populations increase, so do populations of deer ticks and the opportunities for transfer of Lyme disease to humans. Lyme disease could never be maintained in human populations, but excessive deer populations and the proximity of deer to humans involved in outdoor activities have created a significant health hazard in many areas. See also: Lyme disease
Much less certain are the impacts of anticipated global climate change (caused by the burning of fossil fuels, among other factors) on diseases. For example, there is clearly cause for concern about the expansion of tropical diseases into temperate regions that may face significant temperature increases in the future. See also: Global climate change