Key Concepts
A form of energy transfer through conduction, convection, and/or radiation. Heat transfer occurs any time there is a temperature difference between two objects and occurs in the direction of decreasing temperature, meaning from a hot object to a cold object. Although the mechanisms and laws governing the three modes of heat transfer are quite different, they can co-occur in commonplace processes such as boiling a pot of water over an open flame (Fig. 1). This article provides introductory information on heat transfer and on important industrial devices called heat exchangers. See also: Heat
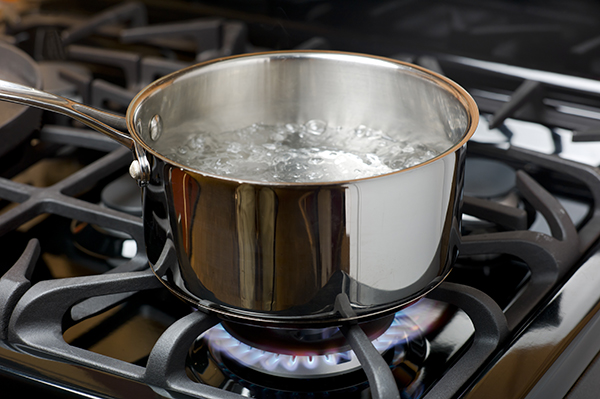
Conduction
Heat conduction involves the transfer of heat from one molecule to an adjacent molecule as an inelastic collision in the case of fluids, as oscillations in solid nonconductors of electricity, and as motions of electrons in conducting solids such as metals. (Note that the term “fluids” includes both liquid and gas phase materials.) For example, heat is transferred by conduction from a hot cooking utensil to your hand, from a soldering iron to the solder, and from the roof of your house to the snow on the roof. Conduction is the only mechanism for the transfer of heat through an opaque solid. Some heat may be transferred through transparent solids, such as glass, quartz, and certain plastics, by radiation. In moving fluids, conduction is supplemented by convection, and if the fluid is transparent, by radiation. See also: Collision (physics); Conduction (electricity); Electricity; Electron; Glass; Metal; Quartz
Conduction heat transfers can be quantified using Fourier’s Law, which includes the important thermal physical property thermal conductivity. The thermal conductivity of a material is an indication of how effectively the material transfers heat. The thermal conductivities of materials vary widely, and thermal conductivity is highest for metals, lower for nonmetals, still lower for liquids, and lowest for gases. Any material which has a low conductivity may be considered to be a thermal insulator. Solids that have high conductivities can be used as thermal insulators if they are distributed in the form of granules, powders, fibers, or foam. Using dispersed materials like foams increases the path length for heat transfer and at the same time reduces the effective cross-sectional area of heat transfer, both of which decrease the heat transfer. Mineral wool, glass fiber, diatomaceous earth, glass foam, Styrofoam, corkboard, Celotex, and magnesia are all examples of such materials. Other applications use high thermal conductivity materials to increase the rate of heat transfer, such as thermal adhesives used to attach fins to microchips. A high thermal conductivity adhesive increases the heat transfer from the hot chip to the fins, and the fins increase the area for convection heat transfer. See also: Heat conduction; Heat insulation
Convection
Like conduction, heat transfer by convection is due to molecular motion. However, during convection molecules are also transported, or moved, due to fluid motion. Convection is quantified using Newton’s Law of Cooling, which includes the heat transfer coefficient. Like the thermal conductivity, the heat transfer coefficient is a function of thermophysical properties of the fluid; however, it is also a function of the physical characteristics of the system, such as the velocity of the fluid and the shape of the flow field. Diffusion is when molecules are transported due to gradients in the fluid (such as temperature gradients). Advection is when molecules are transported by bulk motion of the fluid, such as water flowing in a river. Diffusion and advection can occur simultaneously in a fluid, such as when the water in a river is colder at the bottom of the river and hotter on top. When a fluid is in contact with a surface at a temperature different from the fluid temperature, conduction will transfer heat from the hot surface to the cold fluid (or from a cold surface to a hot fluid). The motion of the fluid will then transfer the heat within the fluid. Continuing with the river example, conduction will occur from the hotter soil at the bottom of the riverbed to the cooler water in the river. Convective heat transfer between a surface and a fluid cannot occur without conduction at the interface between the fluid and the surface. The motion of the fluid may be entirely due to differences in density (resulting from temperature differences in the fluid), as in natural convection (also called buoyant convection), or due to mechanical means (such as a fan), as in forced convection. See also: Density
Convection heat transfer is vital to an enormous number of engineering applications and systems found in nature. For example, most of the heat supplied to a room from a steam or hot-water radiator is transferred by convection. The cooling and heating provided in a vehicle for the passenger compartment is predominantly due to convection heat transfer. The heat from the combustion of natural gas in a hot water heater to the water tank is by convection. Water in lakes and ponds freeze first on the surface and not at the bottom due, in part, to natural convection. Ice cools a drink in a glass, in part, by convection, and soup is heated in a pot by forced convection if the soup is stirred and by natural convection if the soup is not stirred. See also: Heat convection
Heat transferred by convection can involve single-phase fluids (for example, gases or liquids) or fluids that undergo a phase change. Phase changes can dramatically increase the heat transfer due to the additional energy required to vaporize or condense a fluid, for instance via the latent heat of vaporization, solidification, sublimation, or crystallization. As another example, the human body can be cooled to lower than ambient temperature by evaporation of sweat from the skin. Dry ice (solid phase carbon dioxide) can absorb large quantities of heat by sublimating the carbon dioxide. Heat extracted from the products of combustion in a boiler is transferred through a metal tube wall and converts the water inside the tube from liquid to vapor steam. See also: Dry ice; Phase transitions; Skin; Sweat gland; Temperature; Water
Radiation
All materials, regardless of temperature, emit radiation in all directions. Transfer of energy by radiation is unique in that no conducting substance is necessary, as is required with conduction and convection. In other words, radiation heat transfer can occur in a vacuum. This unique property makes possible the transfer of energy from the Sun to the Earth. Radiation heat transfer is quantified by the Stefan-Boltzmann Law and can be interpreted using wave theory, in the same way as light. Radiation heat transfer occurs by interaction of surfaces at different temperatures, and radiation can be absorbed, reflected, or transmitted. The properties that characterize effectiveness at emission, absorption, transmission, and reflection are a function of the surface of the material and are described by the material emissivity, absorptivity, transmissivity, and reflectivity. The net energy transferred by radiation is equal to the difference between the radiation emitted and the radiation absorbed by the surface.
Radiation heat transfer has spectral characteristics, meaning that radiation is a function of wavelength. Radiation from solids is typically a continuous spectrum that spans many wavelengths (for instance, from the visible to the infrared regions). As the temperature of the object increases, the radiation emission shifts to shorter wavelengths associated with higher energies. For example, as the temperature of an iron bar is raised to about 800 K (approximately 525°C or 1000°F), the radiation from the iron shifts from long infrared regions, which are invisible to the human eye, to the visible wavelength region and the iron appears to glow dark red. As the temperature is increased further, the intensity of the radiation increases and the color appears more orange/yellow. This process is also apparent in the filament of an incandescent light bulb. When the bulb is operated at a lower voltage, the light emitted from the filament appears red. As the voltage is increased, the filament temperature increases and the light appears progressively more yellow. See also: Blackbody; Light; Photon; Volt-ampere
Liquids and gases absorb and emit radiation with more distinct spectral characteristics. In other words, liquids and gases can be transparent to some wavelengths of radiation and highly absorptive at other wavelengths. A key example is molecular oxygen (O2) present in the Earth’s atmosphere. The O2 in the Earth’s atmosphere absorbs nearly 100% of the harmful radiation from the Sun in the vacuum ultraviolet (<200 nm). Similarly, greenhouse gases such as carbon dioxide and methane are effective infrared absorbers and responsible for global warming. Many liquids, especially organic liquids, have selective absorption bands in the infrared and ultraviolet regions. See also: Absorption of electromagnetic radiation; Earth; Global climate change; Heat radiation; Nuclear explosion; Sun
Design considerations
The principles governing the three modes of heat transfer can be used to design and analyze systems. Proper selection and fabrication of materials can allow heat transfer for specific purposes, including cooling or heating of specific regions for steady or transient conditions. All three modes of heat transfer can be applied in one process, and conduction, convection, and radiation are commonplace in many familiar systems. In summer, the roof on a house becomes hot because of radiation from the Sun, even though a cool breeze is transferring some of the heat away from the roof by convection. At the same time, conduction transports some of the heat through the roof where it is distributed to the attic by convection. The prudent householder attempts to reduce the heat transferred to the attic by reducing the heat that is absorbed by the roof by painting the roof white. The householder also installs insulation to the underside of the roof to reduce the transfer of heat through the roof. Further, the heated air in the attic can be vented through louvers in the roof.
While all three modes of heat transfer may be present, one mode may dominate the total heat transfer. Importantly, convection and conduction heat transfer rates are both proportional to the temperature difference between the objects of interest. Radiation heat transfer is proportional to temperatures to the fourth power. This non-linear dependence on temperature often causes radiation to surpass conduction and convection heat transfer rates in high temperature systems such as fire and combustion applications. Additionally, radiation is the only mode of heat transfer present in vacuum systems.
Heat exchangers
Heat transfer is a goal in many industries. Devices used for the purpose of transferring heat between two substances are called heat exchangers. Some of the most prevalent types of heat exchangers use two working fluids that are physically separated by a heat-exchange surface in the form of plates or tubes. A car radiator, hot-water heater, steam or hot-water radiator, steam boiler, condenser and evaporator on a refrigerator or air conditioner, and even the ordinary cooking utensils in everyday use are all heat exchangers. See also: Heat exchanger
In power plants, oil refineries, and chemical plants, two commonly used designs are tube-and-shell and double-pipe heat exchangers. The first consists of a bundle of tubes inside a cylindrical shell. One fluid flows inside the tubes and the other fluid flows between the tubes and the shell. The double-pipe heat exchanger consists of one tube inside another tube with one fluid flowing inside the inner tube and the other flowing in the annular space between tubes. In both cases, the tube walls serve as the heat-exchange surface. Heat exchangers consisting of spaced flat plates with the hot and cold fluids flowing between alternate plates are also in use. Each of these exchangers essentially depends upon convection heat transfer through the fluid on each side of the heat-exchange surface and conduction through the surface. Countless special modifications, often also utilizing radiation for heat transfer, are employed for a variety of purposes.
In these exchangers, the fluid streams may flow parallel concurrently or in mixed flow. In most cases, the temperatures of the various streams remain essentially constant at a given physical location, and the process is said to be a steady-state process. As the fluids move through the heat exchangers, unless there is a phase change, the fluids are continuously changing in temperature, and the temperature gradient from one stream to the other may be continuously varying. To determine the amount of heat exchange surface needed for a given process, the designer must evaluate the effective temperature gradient for the particular conditions and particular heat exchanger design.
Often the heating or cooling of an object is desired. In this case, the object does not remain at a constant temperature, and such a process is an unsteady, transient, or time-varying system. The heating or cooling of food in ovens and refrigerators, respectively; the heating of steel billets in metallurgical furnaces; the heating of bricks in a kiln; and the calcination of gypsum are all examples of transient processes.