Key Concepts
The set of anatomical structures (muscle cells, contractile elements, and associated connective tissues) that are responsible for body movements in vertebrates. The muscular system (Fig. 1) is necessary for movements of the vertebrate body. Specifically, the muscular system consists of muscle cells, the contractile elements with the specialized property of exerting tension during contraction, and associated connective tissues. The three morphologic types of muscles are voluntary muscle, involuntary muscle, and cardiac muscle. The voluntary, striated, or skeletal muscles are involved with general posture and movements of the head, body, and limbs. The involuntary, nonstriated, or smooth muscles are the muscles of the walls of hollow organs of the digestive, circulatory, respiratory, and reproductive systems, and other visceral structures. Cardiac muscle is the intrinsic muscle tissue of the heart. See also: Circulation; Digestive system; Heart (vertebrate); Muscle; Muscle development and regeneration; Muscular system disorders; Reproductive system; Respiratory system

The vertebrate muscular system is a large organ system, making up 35–40% of the body weight in humans. The movement of vertebrates is accomplished exclusively by muscular action, and muscles play the major role in transporting materials within the body. Muscles also help to tie the bones of the skeleton together and supplement the skeleton in supporting the body against gravity. See also: Skeletal system
Comparative anatomy
Phylogenetically speaking, muscles are very plastic organs. They have undergone considerable change during the evolution of vertebrates, correlated in large part with changes in the organisms' environments and in their methods of support and locomotion. Establishment of homologies among muscles is not easy. Adult relationships can be misleading because muscles have subdivided during their evolution, and parts have migrated far from their original positions. Nerve supply is a more reliable criterion because nerves have tended to follow the muscles through their evolutionary gymnastics, but often homologies cannot be established without recourse to embryonic development. Determination of the development of the thousands of individual muscles among the vertebrate classes is a monumental task. See also: Animal evolution; Nervous system (vertebrate); Vertebrata
Muscle groups are particularly distinct in elasmobranchs (sharks, skates, and rays) and other primitive fishes, and they are generally defined on the basis of their embryonic origin in these animals. Two major groups of skeletal muscles are recognized: somatic (parietal) muscles, which develop from the myotomes (embryonic muscle blocks or plates that will differentiate into muscle segments); and branchiomeric muscles, which develop in the pharyngeal wall from the lateral plate mesoderm (middle germ layer). The somatic musculature is subdivided into axial muscles, which develop directly from the myotomes and lie along the longitudinal axis of the body, and appendicular muscles, which develop within the limb bud from mesoderm derived phylogenetically as buds from the myotomes. See also: Elasmobranchii
Axial musculature
The axial musculature consists of the muscles of the trunk and head (Fig. 2). Axial musculature located along the back and flanks of the body is referred to as trunk musculature. Anteriorly, though, the axial musculature is modified and assigned to other subgroups. Certain of the occipital and neck myotomes form the hypobranchial muscles, and the most anterior myotomes form the extrinsic ocular muscles.

The epaxial (dorsal) musculature remains powerful in most cases. In mammals, there is a union of various muscles to form a powerful erector spinae (sacrospinalis) complex that helps to support the vertebral column. In addition, the body is held off the ground by the limbs. Much of the epaxial musculature functions as tie members that resist tension stresses along this girder. Anteriorly, there is a cleavage of the epaxial divisions into a host of muscles associated with complex head and neck movements.
The hypaxial (ventral) musculature of tetrapods can be subdivided into three groups: (1) a subvertebral (hyposkeletal) group located ventral to the transverse processes and lateral to the centra of the vertebrae, (2) the flank muscles forming the lateral part of the body wall, and (3) the ventral abdominal muscles located on each side of the midventral line. The subvertebral musculature assists the epaxial muscles in the support and movement of the vertebral column. Most of the flank musculature takes the form of broad, thin sheets of muscle that form much of the body wall and support the viscera. The midventral hypaxial musculature in all tetrapods consists of the rectus abdominis, which is a longitudinal muscle on each side of the midline that extends from the pelvic region to the anterior part of the trunk.
The hypobranchial musculature extends from the pectoral girdle forward along the ventral surface of the neck and pharynx to the hyoid arch and chin, and into the tongue. It is regarded as a continuation of part of the hypaxial trunk musculature.
The extrinsic ocular muscles develop from the prootic somites (head cavities). All vertebrates have six in common. The median (internal), superior, and inferior recti, together with the inferior oblique, develop from the first somite and are innervated by the oculomotor nerve. The superior oblique and lateral (external) rectus develop from the second and third somites, respectively, and are supplied, respectively, by the trochlear and abducens nerves. All of these muscles insert on the eyeball and move it. In addition, most terrestrial vertebrates, with the exception of birds, have a retractor oculi (a cone-shaped muscle lying deep to the recti) that pulls the eyeball deeper into its socket. A levator palpebrae superioris, present in mammals, completes the ocular group; this muscle elevates the upper eyelid in opposition to the action of certain facial muscles. See also: Eye (vertebrate)
Appendicular musculature
Appendicular muscles move the upper and lower limbs. Limb muscles are often classified as intrinsic if they lie entirely within the confines of the appendage and girdle, and extrinsic if they extend from the girdle or appendage to other parts of the body. This scheme has certain merits, but is misleading from the phylogenetic point of view, particularly because the extrinsic muscles are not all appendicular in the sense in which this group has been defined. Some are appendicular muscles that have developed directly from mesoderm in the limb bud, but others are trunk, hypobranchial, and branchiomeric muscles that have secondarily become associated with the girdle.
In fishes, the paired fins are primarily horizontal stabilizing keels, but they also are used in deceleration and steering, and to help control the depth at which the fish swims. Fin movements are not complex or powerful, and the appendicular muscles in the strictest sense are morphologically simple.
In terrestrial vertebrates, the limbs become the main organs for support and locomotion, and the appendicular muscles become correspondingly powerful and complex. The muscles can be sorted into dorsal and ventral groups because tetrapod muscles originate embryonically from dorsal and ventral premuscular masses within the limb bud. In general, the ventral muscles, which also spread onto the anterior surface of the girdle and appendage, act to protract and adduct the limb and to flex its distal segments; the dorsal muscles, which also extend onto the posterior surface of the girdle and appendage, have the opposite effects (retraction, abduction, and extension). The limb muscles also serve as flexible ties or braces that can fix the bones at a joint and support the body.
Flight in birds has entailed a considerable modification of the musculature of the pectoral region. As one example, the ventral adductor muscles are exceedingly large and powerful, and the area from which they arise is increased by the enlargement of the sternum and the evolution of a large sternal keel. Not only does a ventral muscle, the pectoralis, play a major role in the downstroke of the humerus, but a ventral muscle, the supracoracoideus, is active in the upstroke as well. See also: Aves
Integumentary musculature
In a number of terrestrial vertebrates, particularly amniotes, certain of the more superficial skeletal muscles of the body have spread out beneath the skin and inserted into it. These may be described as integumentary muscles; again, though, they are not a natural phylogenetic group, but are derived from several different groups. Integumentary muscles are particularly well developed in mammals and include the facial muscles, platysma (a subcutaneous muscle of the neck), and often a large cutaneous trunci.
Histology
Three histological types of muscle are recognized: smooth, striated (skeletal), and cardiac. Smooth and cardiac muscle fibers generally occur in layers in the walls of organs, but striated muscle fibers are usually grouped into distinct entities, that is, the skeletal muscles of gross anatomy. In some vertebrates, including the rabbit, red and white skeletal muscles can be distinguished; however, in most vertebrates, an individual muscle contains a variable mixture of red and white fibers (Fig. 3). Red fibers contain more sarcoplasm and myoglobin (a muscle protein) than white fibers. Myoglobin has a greater affinity for oxygen than hemoglobin; hence, oxygen can be taken from the blood and stored by red muscle cells. The contraction of red fibers is more sustained, less subject to fatigue, and often slower than the contraction of white fibers. Red muscles, or muscles containing a preponderance of red fibers, tend to be found in situations where the muscles are particularly active in either moving the body or maintaining posture. Some examples are the diaphragm and other respiratory muscles, and the gluteus maximus. White muscles, or muscles with a preponderance of white fibers, are associated with a more intermittent and often a faster and more powerful movement. The biceps and digital muscles are examples. See also: Muscle proteins
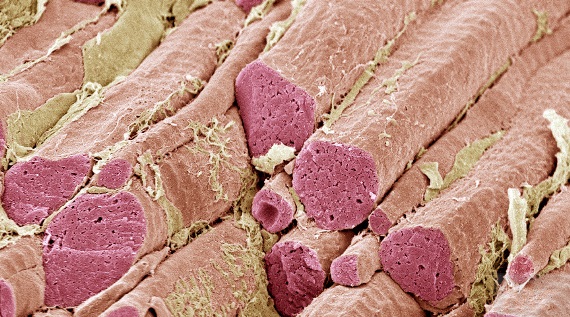
Each skeletal muscle is individualized by a connective tissue sheath, the epimysium, which is a part of the deep fascia, and by its distinctive attachments onto skeletal elements. Skeletal muscles vary greatly with respect to the number of fibers that they contain and in the length and arrangement of the fibers. Important determinants of muscle architecture are the extent and nature of the movement that is to be brought about, the force that must be exerted, and the space available for the muscle in a particular area. The extent to which a muscle shortens is a function of the length of its fibers. Experimentally, muscle fibers can shorten to about one-half of their resting length, but they attach to bones so close to the joints that they seldom shorten this much. The strength of a muscle, on the other hand, is approximately proportional to the number of fibers that it contains.
Muscles are usually arranged so that one muscle or group of muscles will pull a structure in a certain direction, and an opposing muscle or group will pull the structure in the opposite direction. Several sets of terms describe these antagonistic actions. Flexion is the movement of a distal part of an appendage toward a more proximal part; this occurs at the elbow and knee. It also describes the bending of the head or trunk toward the ventral surface. Extension is the opposite movement. Flexion and extension are sometimes also applied to forward and backward movements of the appendage at the shoulder and hip; however, to avoid confusion, the terms protraction for a forward movement and retraction for a backward movement are more appropriate. Abduction is the movement of a part away from some point of reference, and adduction is movement toward it. For the appendages, the reference point is the midventral line of the body. Finally, various types of rotary movements occur. For example, rotation of the bones of the forearm so that the palm of the hand faces up is supination; the opposite movement is pronation.
Muscle mechanics
Many of the bones serve as lever arms, and the contractions of muscles are forces acting on these arms (Fig. 4). The joint, of course, is the fulcrum and it is at one end of the lever. The length of the force arm is the perpendicular distance from the fulcrum to the line of action of the muscle; the length of the work arm is the perpendicular distance from the fulcrum to the point of application of the power generated in the lever. Compactness of the body and physiological properties of the muscle necessitate that a muscle will attach close to the fulcrum; therefore, the force arm is considerably shorter than the work arm. Most muscles are at a mechanical disadvantage because they must generate forces greater than the work to be done; however, an advantage of this is that a small muscular excursion (the distance that a muscle fiber can shorten) can induce a much greater movement at the end of the lever. See also: Biomechanics

Slight shifts in the attachments of a muscle that bring it toward or away from the fulcrum, and changes in the length of the work arm, can alter the relationship between the force and amount of speed of movement. In general, the force of a muscle is inversely related to the amount and speed of movement that it can cause. Certain patterns of the skeleton and muscles are adapted for extensive, fast movement at the expense of force, whereas others are adapted for force at the expense of speed. For example, in the limb of a horse, which is adapted for long strides and speed, the muscles that move the limb insert close to the fulcrum and the appendage is long. This provides the lever system with a short force arm, but a very long work arm (Fig. 4). In contrast, in the front leg of a mole, which is adapted for powerful digging, the distance from the fulcrum to the insertion of the muscles is relatively greater and the length of the appendage is less, with the result that the length of the force arm is increased relative to the length of the work arm.
Embryology and histogenesis
Embryonic muscle cells are known as myoblasts. Most muscles are derived from mesoderm (the middle germ layer); the exceptions are the sphincter and dilator muscles of the iris and the myoepithelial cells of the sweat and mammary glands, which are derived from ectoderm (the outer germ layer). The embryonic mesoderm that differentiates into muscle tissues includes the dorsal mesoderm, head mesenchyme, intermediate mesoderm, and lateral mesoderm. The dorsal mesoderm that condenses into bilateral columns adjacent to the neural tube forms the segmentally arranged myotomes. Most intrinsic voluntary muscles of the neck and trunk are differentiated from these myotomes. In the higher vertebrates, the limb muscles are mainly derived from lateral plate mesoderm. In tetrapods, the myoblast component of all truncal and appendicular muscles originates from somites (bilaterally paired blocks of mesoderm). The voluntary muscles of the branchial (visceral) arches of the head and neck are derived directly from head mesenchyme. See also: Embryonic differentiation; Embryonic induction
Smooth muscles arise from mesenchymal cells. During embryonic life, the cells migrate and concentrate in the vicinity of the epithelial linings of the hollow organs. The myoblasts elongate and orient themselves as in the adult organ. Myofibrils (contractile elements) are visible in the early stages and become more numerous during later development.
Cardiac muscle develops from the splanchnic mesoderm of the heart tube. The myoblasts adhere to each other. However, unlike skeletal muscle, the plasma membranes do not disintegrate; rather, the sites of adhesion give rise to the intercalated discs (gap junctions). As in skeletal muscles, myofibrils and the other structural muscle elements differentiate early.