The process that led to the formation of the continents. The Earth's crust is distinctively bimodal in thickness. Oceanic crust is normally about 4 mi (7 km) thick, varying mainly with the temperature of the mantle beneath the sea-floor spreading ridges when the crust was formed. In contrast, the typical 22–25-mi (35–40-km) thickness of continental crust is controlled ultimately—through the agents of erosion, sedimentation, and isostatic adjustment—by sea level. Oceanic crust is formed at spreading ridges, continental crust at subduction zones. Both are recycled to the mantle, but oceanic crust, being less buoyant, is recycled about 30 times faster than continental crust. Consequently, continents, having a mean age of almost 2 billion years and a maximum age of 4 billion years, provide the only directly accessible record spanning most of Earth history. They are, however, structurally more complex than ocean basins because of their great antiquity and weak rheology. See also: Earth crust; Mid-Oceanic Ridge
Constructive processes
Subduction zones are the main factories for making continental crust (Fig. 1). The primary constructive processes are trench accretion, arc magmatism, and arc-continent collision. Mantle plumes and lithospheric stretching cause secondary magmatic additions to continental crust. See also: Lithosphere; Magma; Subduction zones
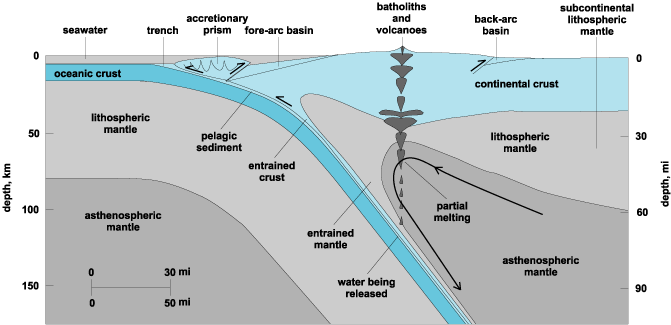
Trench accretion
Where an oceanic plate sinks beneath a continental plate or another oceanic plate, sediment scraped off the top of the descending plate accumulates as an accretionary prism at the leading edge of the overriding plate (Fig. 1). Accretionary prisms are bounded by trenches on one side and fore-arc basins on the other. They are best developed at trenches containing thick accumulations of terrigenous sediment, where the off-scraping process is facilitated by high fluid pore pressures in the sediment. Accretionary prisms may also contain slices of pelagic ooze and underlying oceanic crust, volcanic seamounts and their capping atoll reefs, or far-traveled deep-sea fans. They are typically dismembered: accretion does not proceed smoothly because the top of the descending plate is subject to block faulting related to the bending of the plate. The accreted material is dewatered, deformed, and metamorphosed as the accretionary prism grows. Accretionary prisms are rarely active magmatically. Where they intersect spreading ridges, the descending oceanic crust may be hot enough to partially melt at relatively shallow depths. A distinctive suite of sodic granitoid intrusive rocks record such events. Trench accretion is dominantly a process of crustal reworking, not crustal growth, because the bulk of the sediment is derived from the erosion of preexisting crust. See also: Atoll; Reef
Arc magmatism
A volcanic arc is a surface manifestation of partial melting near the tip of the mantle wedge above the subducting slab (Fig. 1). Melting in the wedge is induced by the infiltration of aqueous fluids, which lower the temperature required for melting to begin (hydration melting). The fluids are derived from the breakdown of hydrous minerals associated with the subducting oceanic crust. Depth-dependent release of water controls the location of the volcanic front facing the trench, typically 60–70 mi (100–120 km) vertically above the slab. A fertile source of melting in the mantle wedge is maintained by convective flow driven by the viscous drag of the cold descending slab. Although the main source of arc magmas is the mantle wedge, sediment and ablated crust entrained in the slab make subordinate contributions to arc magmas. The mantle-derived fraction represents new crustal addition. The slab-derived melts serve to speed up (fast-track) the mantle recycling process.
Unlike mid-ocean ridges, magmatic arcs evolve with time because the supply of magma is not balanced by crustal spreading. The crust of volcanic arcs thickens through the addition of new magma. Consequently, the compositions of the magmas evolve as the height of the mantle melting column decreases and the filtering effect of the overlying crust increases. The filtering effect causes crustal differentiation with more buoyantly mobile components concentrating upward. Denser and more refractory components collect in the lower crust. The magmas ingest and assimilate wall rocks and interact with meteoric groundwaters, producing hybrid, compositionally diverse, igneous rock suites. Moreover, magmatic arcs are usually the rheologically weakest part of the lithosphere in subduction zones because of their high heat flow. Consequently, they localize tectonic deformation, which generally combines wrenching parallel to the trench (driven by oblique subduction) and extension or compression across the arc (governed by changes in buoyancy of the subducting slab or motion of the upper plate). See also: Igneous rocks
Collisions
Where subduction occurs beneath continental lithosphere, trench accretion and arc magmatism add crust to the continent directly. Continental-type protocrust is also formed at subduction zones (often having complex developmental histories) situated entirely within oceanic lithosphere. Incorporation of such protocrust in a continent is accomplished by arc-continent collision (Fig. 2). The reversal of subduction polarity that typically follows arc-continent collision (best exemplified in the modern world by the diachronous collision—a collision progressing from north to south with time—at Taiwan between the Luzon arc of the Philippines and southeast China) is the principal means by which subduction beneath a continent is initiated.
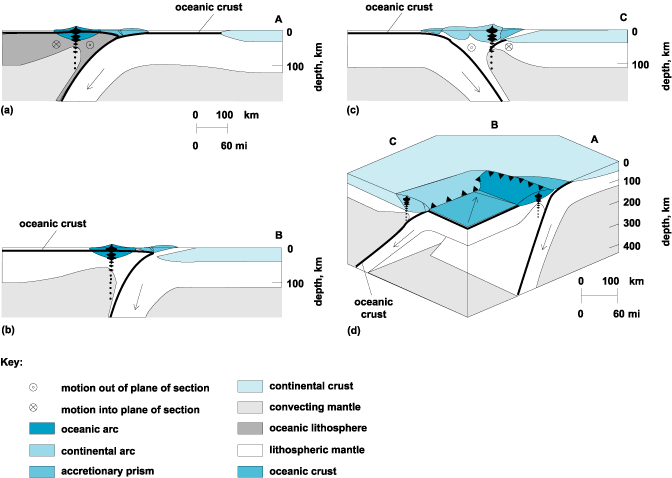
Final closure of an ocean basin results in continent-continent collision, a mainly destructive event. Tectonic crustal thickening produces mountains and greatly accelerated rates of erosion. Much of the resulting sediment is washed off the continent onto deep-sea fans or trenches, and some fraction of that sediment will be subducted. Small crustal gains may be made if the tectonically thickened lithospheric mantle becomes unstable and sinks away. The hotter replacement mantle may partially melt as it rises to take its place (decompression melting), causing small magmatic additions to the already thickened continental crust. See also: Basin; Orogeny
Plumes and rifts
Plumes are jets of anomalously hot mantle that partially melts as it reaches the lithosphere, causing the volcanism of Hawaiian-type islands and related seamount chains. Plumes also cause volcanism on continents, for example, Yellowstone in North America. Starting plumes have large heads and relatively small tails through which the plume is maintained. The arrival of starting plume heads at the lithosphere is signaled by continental flood basalt or oceanic plateau volcanism. The largest volumes of melt are generated where lithospheric stretching (upper crustal rifting) occurs in conjunction with a plume. The combination maximizes the potential for decompression melting. In addition to surface volcanism, the melts may pond at or near the base of the crust, causing magmatic underplating. Seamounts and oceanic plateaus are fragmentarily accreted to continents at trenches. See also: Basalt; Oceanic islands; Seamount and guyot; Volcanology
Destructive processes
Subduction zones are sites of continental accretion, but they may also cause destruction of continental crust (Fig. 1). The destructive processes are sediment subduction and subduction ablation. Constructive and destructive processes may be at work simultaneously, and the net balance may swing one way or the other with time. Selective destruction of lower crust may occur in continent-continent collision zones as a result of convective dripping of tectonically thickened lithosphere.
Sediment subduction
Accretionary prisms are poorly developed or lacking where trenches receive only very sparse amounts of terrigenous sediment. In such cases, pelagic oozes are subducted along with the oceanic crust. Even at subduction zones with well-developed accretionary prisms, some of the pelagic and terrigenous sediments disappears beneath the deformation front of the prism. Some is accreted structurally to the base of the prism, and some is transferred to the magmatic arc by melting. Some escapes melting and sinks deeply into the mantle, where it constitutes an isotopically recognizable component in the source of plume basalts. Sediment subduction, being fed by surface erosion, preferentially destroys the upper crust.
Subduction ablation
It is postulated that crust and lithospheric mantle from the overriding plate may become entrained in the subducting slab, causing tectonic ablation. Like subducted sediment, ablated crust is potentially capable of melting as it passes beneath the convecting mantle wedge. Unlike subducted sediment, ablated material may include lower crust and lithospheric mantle as well as upper crust. The ablation flux is quantitatively unknown.
Continental drip
Where continental lithosphere is tectonically thickened, cold lithospheric mantle is forced downward into hotter convecting mantle. Lithospheric thickening generates lateral thermal gradients, which drive mantle convection. The thickened lithospheric mantle is therefore unstable and may drip away. The missing lithospheric mantle is replaced by hotter asthenospheric mantle, causing melting and surface uplift. Lower crust may be entrained in the drip if it has been transformed to eclogite (a high-density rock chemically equivalent to basalt but composed of garnet and pyroxene) under the load of the tectonically thickened crust. Unlike sediment subduction, upper crust is not lost by dripping; and unlike subduction ablation, continental drips are not susceptible to hydration melting. Drips may sink deeply into the mantle; this view is based on the recognition of distinctive chemical and isotopic signatures of subcontinental lithospheric mantle in plume basalts. See also: Asthenosphere; Eclogite
Continents and Earth history
The oldest surviving crust on Earth was formed 4 billion years ago. The absence of crust older than 4 billion years is attributed to recycling associated with the early high-impact flux inferred from the dated cratered surface of the Moon. Since the impact flux waned, processes that construct and destroy continental crust have adjusted to slow cooling and convective slowing of the mantle. The estimated magnitude of the secular change in mean upper-mantle temperature since 4 billion years ago is on the order of 360°F (200°C). This is comparable in magnitude to regional temperature variations in the upper mantle at the present time. In effect, mantle plumes in the present simulate more general conditions that prevailed in the early Earth. Furthermore, processes limited to the earlier or later parts of Earth history should be only those that operate in anomalous situations. This reasoning is consistent with empirical comparisons of continental crust of different ages. The basic forms and scales of continental accretion as well as those of continental fragmentation and collision appear to have changed remarkably little over geologic time. See also: Moon
Oldest crustal relics
Areas of crust between 3.5 and 4 billion years old are small (less than 390 mi2 or 1000 km2), and there is nothing extraordinary about the rock suites they contain. Their isotopic characteristics and variability are striking. They show that the contemporaneous mantle was very heterogeneous, containing different sectors strongly depleted in some crustal components and strongly enriched in others. The crust was also heterogeneous and included sectors having long crustal residence times (greater than 200 million years) as well as newly formed sectors. These observations suggest that abundant continental-type crust already existed and that it was subject to vigorous recycling. However, these inferences are widely disputed. See also: Rock age determination
Early juvenile crust
Juvenile crust, in a strict temporal sense, is continental crust recently separated from the mantle. In the broader nontemporal sense used here, it is continental crust not strongly modified by postaccretionary tectonism. Coherent areas up to 1550 mi (2500 km) in diameter of juvenile crust older than 2 billion years are exposed in Precambrian shields. Although rocks and structures typical of accretionary prisms, magmatic arcs, and fore-arc and back-arc basins abound, some systematic differences in comparison with geological younger juvenile crust are evident. The relative abundance of certain sodic granitoid suites in old shields implies a secular decline in the importance of slab (crustal) melting relative to wedge (mantle) melting in subduction zones. Komatiites (extremely hot, magnesium-rich, lava flows) are essentially confined to old shields and are interpreted as representing mantle-plume volcanism in the early Earth. Conversely, blueschists (rocks metamorphosed at high pressure and low temperature) are unknown in old shields. They are products of extremely rapid burial and exhumation in subduction zones having severely depressed geothermal gradients. Blueschists could not have survived even slightly increased geothermal gradients. These and other observations are consistent with the estimated cooling of the Earth and consequent adjustments in the tectonic regime. See also: Blueschist; Precambrian
Cratons and mantle roots
Areas of juvenile crust older than about 2 billion years tend to be rheologically stiffer than younger areas. Such strong blocks are called cratons. Most have survived several rounds of continental fragmentation and collision, their margins battered by repeated openings and closing of ocean basins. Mantle tomography (three-dimensional mantle thermal structure inferred from velocity variations of earthquake-generated seismic waves) shows that cratons are underlain by anomalously cold mantle corresponding to lithosphere more than twice the approximately 60-mi (100-km) thickness of mature oceanic or noncratonic continental lithosphere. The mantle roots must be chemically distinct because, given that cratons tend to have neutral or even positive buoyancy, a low intrinsic density is required to offset the density increase due to thermal contraction. See also: Computerized tomography; Continental margin
Conveniently, rock samples of mantle roots (peridotites) have been delivered to the surface as inclusions in diamond-bearing subvolcanic pipes. The inclusions have appropriate densities and are composed of residual mantle material from which high degrees of partial melt were driven. A class of associated inclusions, called eclogites, consists of rocks corresponding compositionally to oceanic crust but metamorphosed under mantle depth conditions. Isotopic studies reveal that the eclogite inclusions are samples of ancient oceanic crust subducted billions of years ago to depths exceeding 90 mi [150 km (into the diamond stability field)]. There they remained, fixed within drifting lithospheric plates, until fired volcanically to the surface in relatively recent times. If the peridotite and eclogite inclusions are cogenetic, the former should also have originated at sea-floor spreading ridges billions of years ago. See also: Diamond; Peridotite
When the Earth was hotter, greater degrees of melting at oceanic spreading ridges (comparable to modern spreading ridges situated above mantle plumes, for example, Iceland) would have generated oceanic lithosphere characterized by thick (greater than 12 mi or 20 km) crust and highly depleted mantle. Such lithosphere might not sink deeply upon subduction because of the buoyancy of the thick crust and because the removal of melt actually lowers the density of the residual mantle. Accordingly, it is postulated that cratonic mantle roots are composed of buoyantly subducted slabs of highly depleted oceanic lithosphere. The presence of mantle roots would have increased the survivability of cratons: they would have been less susceptible to tectonic thickening and destruction by sediment subduction. Therefore, noncratonic continents may have existed on the young Earth but were selectively destroyed.
Continental growth
The mean age of extant continental crust is about 2 billion years. The primary mode in crustal abundance by geologic age is at 2.7 billion years, and perhaps half of all extant crust formed between 1.5 and 3 billion years ago. The conventional interpretation is that there was little continental crust following the period of high impact flux and that continental growth was slow at first, then rose to a peak 2–3 billion years ago, after which it slowly tapered off. An alternative interpretation, however, holds that the volume of continental crust has been in a near steady state since the impact flux waned. The present age distribution is explained by assuming a secular decline in the rate of crustal recycling, presumably modulated by the decreasing vigor of mantle convection as the Earth cooled. The difference in interpretation hinges on the importance assigned to recycling of continental crust. In crustal growth models, there is little crust older than 3 billion years, because little was formed. In steady-state models, much crust was formed early on but little of it survives. The steady-state interpretation is consistent with isotopic data showing that the oldest crustal relics contain highly evolved as well as juvenile components, and that the contemporaneous mantle was also heterogeneous and included strongly depleted regions. Furthermore, the near steady-state alternative is supported by comparative planetology, which indicates that for the hot young mantle not to be thoroughly differentiated by near-surface melting is physically implausible. See also: Continental drift; Convection in the Earth; Earth; Earth's age; Fault and fault structures; Geophysics; Marine geology; Plate tectonics; Seismology