Key Concepts
A large space telescope optimized for observations in infrared wavelengths through science operations in cryogenic conditions. Designed for addressing a range of astrophysical and cosmological questions, the James Webb Space Telescope, or JWST, in many ways is the scientific and technological successor of the Hubble Space Telescope and the Spitzer Space Telescope. The National Aeronautics and Space Administration (NASA) developed JWST in collaboration with the European Space Agency (ESA) and the Canadian Space Agency (CSA). Northrop Grumman served as the prime contractor for JWST. The telescope, initially called the Next Generation Space Telescope when it began development in 1996, was renamed for a former NASA administrator in 2002. On December 25, 2021, JWST (Fig. 1) launched from French Guiana atop an Ariane 5 rocket. The telescope reached its science destination at the second Lagrangian point (L2) of the Sun-Earth system, located 1.5 million kilometers (940,000 mi) from the Earth in the anti-Sun direction, about a month later. Commissioning was completed in July 2022 and the observatory's first science images were publicly released. See also: Astronomical observatory; Astronomy; Astrophysics; Astronomical imaging; Cosmology; Cryogenics; Hubble Space Telescope; Infrared astronomy; Spitzer Space Telescope
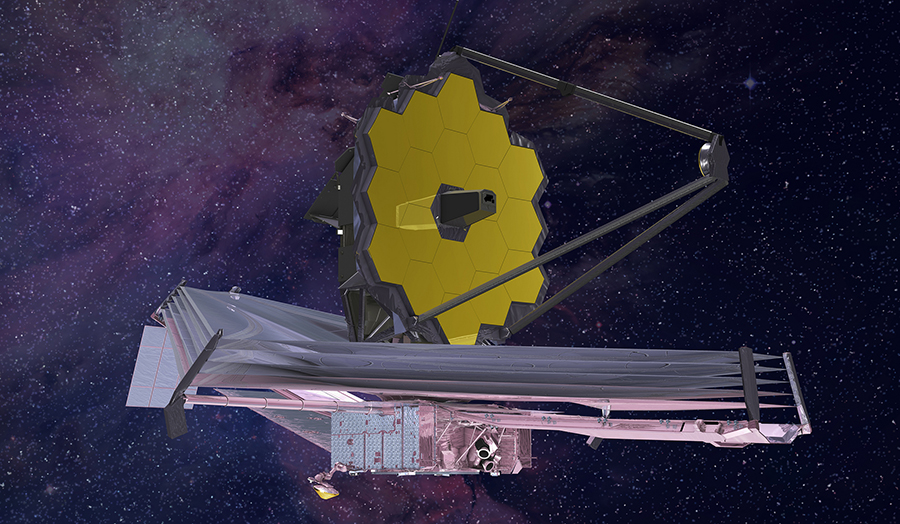
Design
The four main elements of JWST are the Optical Telescope Element (OTE), the Integrated Science Instrument Module (ISIM), the sunshield, and the spacecraft bus (Fig. 2). Multiple innovative technologies were developed for these elements.
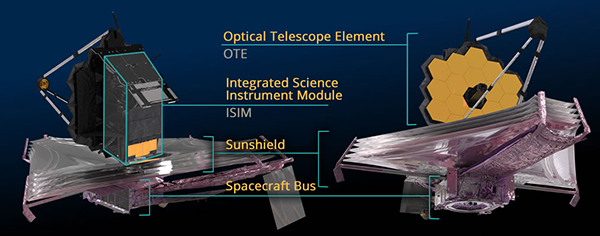
Optical Telescope Element (OTE)
The OTE contains the observatory's large, 6.6-m- (260-in.-) diameter primary mirror, which provides six times the collecting area of the Hubble Space Telescope 2.4-m mirror. Being too large to fit within available rocket fairings, JWST's primary mirror was designed to fold up before launch and redeploy once in space. The mirror itself is formed out of 18 hexagonal-shaped mirror segments, each measuring 1.32 meters (4.3 feet) in diameter. These innovatively lightweight, cryogenic segments weigh approximately 20 kilograms (46 pounds) and are made of the element beryllium, which is both lightweight and strong. The mirrors are coated with highly reflective gold. Small mechanical motors, called actuators, move and change the radius of curvature of the mirror segments for both the primary and secondary mirrors to ensure desired alignment and shape for achieving perfect focus. See also: Beryllium; Gold; Mirror optics; Optics
Integrated Science Instrument Module (ISIM)
The ISIM provides the structure, thermal environment, control electronics, and data handling for JWST's four science instruments. These instruments are:
- Near-Infrared Camera (NIRCam). NIRCam serves as JWST's primary imager in the wavelength range of 0.6–5 μm. Required by many of the core science goals, the instrument is particularly well suited for detecting the first light-emitting objects that formed after the big bang. NIRCam is equipped with a coronagraph in order to obtain images of debris disks, Kuiper Belts, and planets around nearby stars. NIRCam is provided by the University of Arizona.
- Near-Infrared Spectrograph (NIRSpec). The instrument serves as the principal spectrograph in the 0.6–5-μm wavelength range. Spectroscopy is the study of light after it has been separated into its component colors. This technique reveals the composition, temperature, and other physical characteristics of celestial objects. NIRSpec can obtain simultaneous spectra of more than 100 objects in a single observation and is the first space-based instrument to have this multi-object capability. An innovative technology, called a microshutter array, makes these observations possible. The array consists of microshutter cells, each about as narrow as a human hair, that are equipped with lids that can be controlled to toggle open and shut by applying a magnetic field. In this way, the microshutters can block out unwanted light from nearby, foreground objects and allow the instrument to collect more light from faint, distant, background objects. NIRSpec thus enables astronomers to better characterize the early universe and increases the chances of finding rare and unique objects. NIRSpec is provided by ESA with components provided by NASA's Goddard Space Flight Center. See also: Astronomical spectroscopy; Light; Spectroscopy
- Mid-Infrared Instrument (MIRI). MIRI offers imaging and spectroscopy at wavelengths of 5–28 μm. The instrument is intended to study the creation of the first heavy elements and the formation and evolution of galaxies and very old stellar populations. MIRI is uniquely capable of studying the very early stages of star and planet formation in regions where all visible light is blocked by dust and most of the emission is radiated at mid-infrared wavelengths. To best capture this faint warmth, MIRI requires additional cooling than that obtained passively via the sunshield (see next subsection). The additional cooling is obtained through a cryocooler system that utilizes a helium refrigerator, effectively lowering the instrument's detector temperature to 7 K (−266°C or −447°F). MIRI is provided by the European Consortium with the European Space Agency (ESA) and by the NASA Jet Propulsion Laboratory (JPL).
- Fine Guidance Sensor/ Near InfraRed Imager and Slitless Spectrograph (FGS/NIRISS). The FGS enables JWST to point precisely at targets. The NIRISS is intended for the detection and characterization of exoplanets, among other science objectives. The instrument overall has a wavelength range 0.8–5 μm. FGS/NIRISS is provided by the CSA.
Sunshield
To successfully observe faint infrared light, which is the equivalent of faint heat signals, JWST's mirror and science instruments must be kept extremely cold. The sunshield is a major enabler of these cryogenic science operations. The sunshield divides the observatory into a shadowed, cold side for science and a warm side for other spacecraft operations. Accordingly, the sunshield blocks out external sources of light and heat, including the Sun, Earth, and Moon, along with the heat generated by the spacecraft bus. The sunshield is made from a Kapton, a lightweight material with desirable thermal properties, and has five layers separated by the vacuum of space, which is a highly effective insulator and thus reduces the transfer of heat. On the cold side of the sunshield, which faces into deep space, JWST can passively cool to approximately 35 K (−238°C or −397°F) while the warm side reaches approximately 383 K (110°C or 230°F). The sunshield folds up for launch and then deploys to dimensions of 21.197 m by 14.162 m (69.5 ft by 46.5 ft), or roughly the size of a tennis court. See also: Heat; Heat transfer; Temperature
Bus
The spacecraft bus supports the operation of JWST through six major subsystems providing electrical power, attitude control, communications, command and data handling, propulsion, and thermal control.
Science objectives
JWST can see even farther into space and time than is currently possible with the Hubble Space Telescope and other instruments, thus helping to analyze faint sources of light that to date have not been detected. Hubble can see back to about 500 million years after the big bang; JWST is expected to routinely see well past that range and possibly even as far back as 100 million years post-big bang. JWST is designed for observations primarily in infrared wavelengths to capture what initially had been emitted as higher-energy light, in the ultraviolet and visible range, by nascent stars and galaxies early in the history of the universe. This light has traveled so far by the time it reaches the vicinity of Earth that the light has been stretched, or redshifted, into the infrared range. No astronomical tools have previously been able to observe this particular cosmic “dark zone,” but by serving as a “first light machine,” JWST is intended to finally reveal the universe when it was a fraction of its current age and size, when the first stars and galaxies were beginning to form. Of particular interest is resolving the galaxies that host quasars, which are highly active supermassive black holes. See also: Big bang theory; Black hole; Galaxy; Galaxy formation and evolution; Observing quasar-hosting galaxies with the James Webb Space Telescope; Quasar; Redshift; Star
In addition to observing these first stars and young galaxies, mission planners intend for JWST to handle other major objectives over its 5–10-year lifetime. JWST can further determine the geometry of the universe, its age, and its ultimate fate by collecting observations that constrain the properties of dark matter and dark energy. These poorly understood entities comprise about 25 and 70 percent of the universe's mass/energy content, respectively, with the former exerting gravity while the latter acts as a repulsive force accelerating the universe's expansion. See also: Accelerating universe; Dark energy; Dark matter; Gravity; Universe
Although mission planners designed JWST primarily to observe the farthest reaches of the universe, it also can look at objects in the modern universe. With JWST, scientists can study the history of the Milky Way Galaxy and its nearby galactic neighbors by studying the old stars and stellar remnants that formed over our galaxy's lifetime. See also: Milky Way Galaxy
Astronomers also can use JWST to study star birth and formation. The observatory's infrared sensors can pierce the dust and gas that surround stellar nurseries and reveal the processes that dictate the mass and composition of stars, as well as the production of heavy elements. See also: Cosmochemistry; Stellar evolution
Mission planners additionally designed JWST to study the origins and evolution of planetary systems like our own. JWST can study the atmospheres of exoplanets spectroscopically, revealing compositions and characteristics. These investigations are intended to learn more about the potential habitability of worlds, particularly those close to our solar system that are the most amenable to such studies. The observatory's coronagraphs also enable the direct imaging of large exoplanets by blocking out the glare of host stars. See also: Astrobiology; Coronagraph; Exoplanet; Planet
JWST can advantageously observe and gather spectra from objects in our solar system as well, including planets, minor planets such as Pluto and Eris, and small bodies including asteroids, comets, and Kuiper Belt objects. See also: Asteroid; Comet; Kuiper Belt; Pluto; Solar system
Orbital considerations
JWST's science operations take place at L2, one of the five Lagrangian points in the orbital plane of the Earth and Sun at which a third object of negligible mass can remain in equilibrium. The L2 orbit offers a thermally stable environment. At the L2 point, JWST orbits around the Sun rather than the Earth. This arrangement allows JWST to reside in the shadow of its sunshield. As JWST traveled to L2, the telescope's various components deployed, including solar panels for generating electricity, the communications antenna, the sunshield, and the secondary and primary mirrors (Fig. 3). See also: Celestial mechanics; Orbital motion
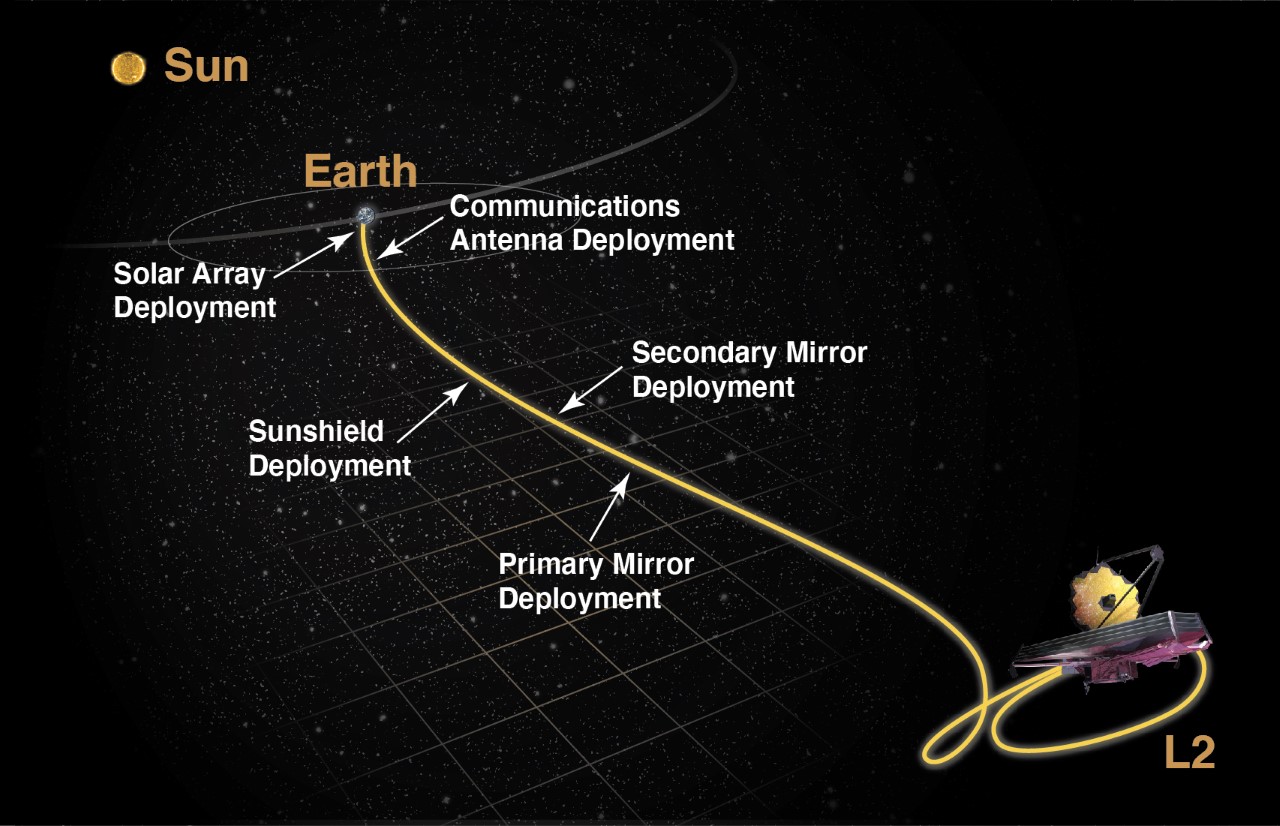