Observing a neutron star in infrared light—a low-energy form of radiation seldom used for studying these cosmic objects—has revealed features that seem to have eluded astronomers' notice going back decades. The features could be a dusty disk encircling the neutron, or a wind of particles streaming off the star into nearby space. In either case, the features would present a newly complex picture of the immediate environment around neutron stars, as well as offer novel ways to investigate their characteristics. See also: Energy; Infrared astronomy; Infrared radiation; Light; Neutron star; Radiation; Star
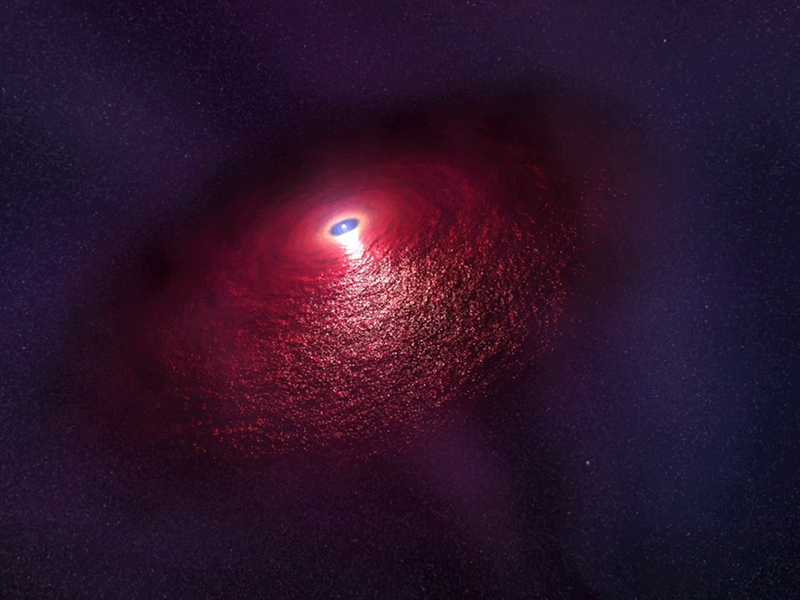
Neutron stars are the small, extremely dense, rapidly rotating remnants of massive stars that have exploded as supernovae. Some neutron stars are hotter than expected, given their observed spin-down rates and inferred ages. Amongst this subset, a research team for the first time detected an emission of isolated infrared light coming from the region offset from a neutron star with the designation RX J0806.4-4123. The Hubble Space Telescope captured this infrared signal, which extends about 200 times the average Sun-Earth distance (known as an astronomical unit) beyond the pulsar source. See also: Astronomical observatory; Astronomical unit; Earth; Hubble Space Telescope; Pulsar; Sun; Supernova; Telescope
Warm, dusty material in space is well-known for producing these sorts of infrared emissions. The researchers therefore suggest that a so-called fallback disk of material might be surrounding the neutron star. This disk would be composed of matter originating in the star that went supernova and left behind the neutron star. A portion of matter could be falling back onto the neutron star, increasing its temperature and, in the process, warming the disk, making it detectable. See also: Matter (physics); Temperature
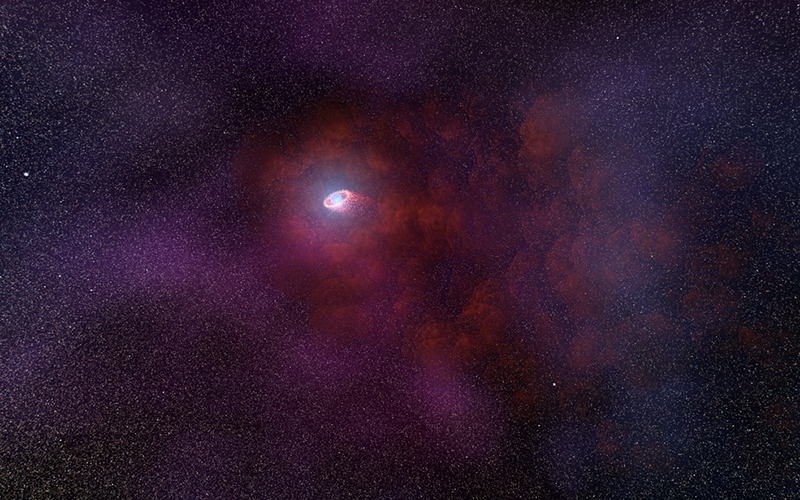
Another possibility is that the neutron star is sending a wind-like gale of particles into space, forming a nebula. A shock wave would build up from these emissions as the neutron star moves through the ambient gases in the galaxy, known as the interstellar medium. Particles in this interaction zone would produce synchrotron radiation, manifesting as the infrared light signature seen by Hubble. See also: Interstellar matter; Nebula; Shock wave; Solar wind; Synchrotron radiation
Overall, the new results illustrate the long-known advantages of studying cosmic phenomena in an array of wavelengths of light, from the highest-energy gamma rays to the lowest-energy radio waves. Astronomers hope that new insight into neutron star evolution will now emerge, giving these objects their scientific due across the entirety of the electromagnetic spectrum. See also: Electromagnetic radiation; Gamma-ray astronomy; Gamma ray; Photon; Radio astronomy