Key Concepts
In common usage, a kind of radiant energy associated with vision, but in the broader sense, the entire range of radiation known as the electromagnetic spectrum. The electromagnetic spectrum is a broad band of energy conveyed by elementary particles, known as photons, which are the force carriers of the electromagnetic force, one of the four fundamental interactions in nature. The spectrum extends over a range of wavelengths running from trillionths of a millimeter to hundreds of kilometers. Arranged in order of increasing wavelength, which equates to lower frequency and decreasing energy, the radiation making up the electromagnetic spectrum is categorized as gamma rays, x-rays, ultraviolet rays, visible light, infrared waves, microwaves, and radio waves (Fig. 1). The wavelengths in the visible light spectrum, which range from about 700 nanometers (nm, billionths of a meter) down to 390 nm, are perceived by the human eye and brain as distinct colors, often roughly categorized—from shorter to longer wavelength—as violet, indigo, blue, green, yellow, orange, and red. See also: Color vision; Electromagnetic radiation; Electromagnetism; Elementary particle; Eye (vertebrate); Fundamental interactions; Photon; Standard model
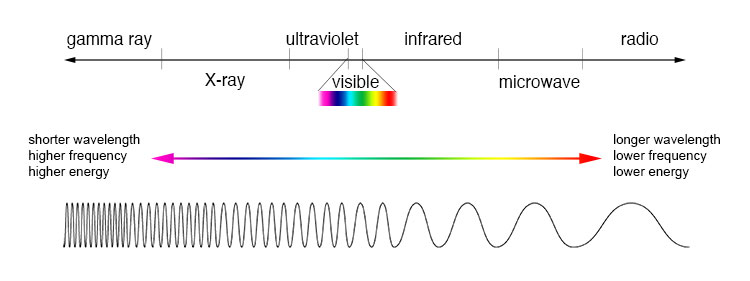
The branch of science dealing with light, its origin and propagation, its effects, and other phenomena associated with it is called optics. Spectroscopy is the branch of optics that pertains to the production and investigation of spectra. See also: Optics; Spectroscopy
Properties of light
Speed of light
Light travels at a finite speed that is considered a universal “speed limit,” with no phenomena in nature able to move faster between two points. In round numbers, the speed of light in vacuum or air may be said to be 300,000 km/s or 186,000 mi/s. Measurements of the speed of light, c, which had attracted physicists for at least three centuries, came to an end in 1983 when the new definition of the meter fixed the value of the speed. Highly precise values of c were obtained by extending absolute frequency measurements into a region of the electromagnetic spectrum where wavelengths can be most accurately measured. These advances were facilitated by the use of stabilized lasers and high-speed tungsten-nickel diodes, which were used to measure the lasers' frequencies. The measurements of the speed of light and of the frequency of lasers yielded a value of the speed of light limited only by the standard of length which was then in use. This permitted a redefinition of the meter in which the value of the speed of light assumed an exact value, 299,792,458 m/s. The meter is defined as the length of the path traveled by light in vacuum during a time interval of 1/299,792,458 of a second. See also: Laser; Length; Standard (physical measurement)
The speed of light is one of the most interesting and important of the fundamental physical constants. It is used to convert light travel times to distance, as in laser and radio measurements of the distance to the Moon and planets. It relates mass m to energy E in German-born U.S. theoretical physicist Albert Einstein's equation, E = mc2. See also: Energy; Fundamental constants; Mass
Diffraction, reflection, and refraction
One of the most easily observed facts about light is its tendency to travel in straight lines. Careful observation shows, however, that a light ray spreads slightly when passing the edges of an obstacle. This phenomenon is called diffraction. See also: Diffraction
The reflection of light is also well known. The Moon, as well as all other satellites and planets in the solar system, are visible only by reflected light; they do not emit visible light like the Sun. However, all celestial bodies emit heat radiation in the infrared spectral range. Reflection of light from smooth optical surfaces occurs so that the angle of reflection equals the angle of incidence, a fact that is most readily observed with a plane mirror. See also: Heat radiation; Moon; Reflection of electromagnetic radiation; Satellite (astronomy); Solar system; Sun
When light is reflected irregularly and diffusely, the phenomenon is termed scattering. The efficiency of light scattering by atoms or molecules increases rapidly with light frequency, reaching its maximum over the visible spectrum in the violet region. The human eye, however, responds differently to different colors according to the three types of color receptors, most strongly to blue light. This explains why the scattering of light by molecules and particles in the atmosphere is seen as the blue color of the sky. When a change in frequency (or wavelength) of the light occurs during scattering, the scattering is referred to as the Raman effect. See also: Raman effect; Scattering of electromagnetic radiation
The type of bending of light rays called refraction is caused by the fact that light travels at different speeds in different media—faster, for example, in air than in either glass or water. Refraction occurs when light passes from one medium to another in which it moves at a different speed. Familiar examples include the change in direction of light rays in going through a prism, and the bent appearance of a straw partially immersed in water (Fig. 2). See also: Refraction of waves
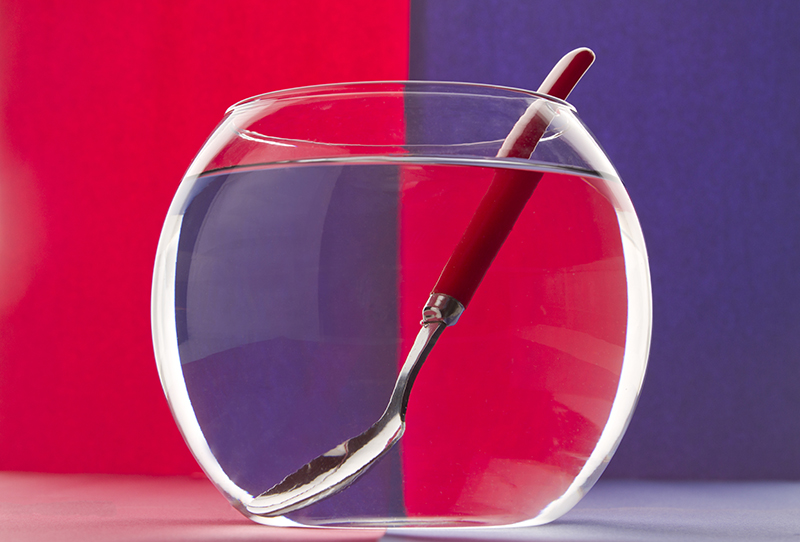
Interference and polarization
In the phenomenon called interference, rays of light emerging from two parallel slits combine on a screen to produce alternating light and dark bands. This effect can be obtained quite easily in the laboratory, and is observed in the colors produced by a thin film of oil on the surface of a pool of water. See also: Interference of waves
Polarization is a property of light that describes the orientation of the electromagnetic wave oscillation. Polarization of light is usually demonstrated by using a polarizer, which transmits light of one polarization. A polarizer can be quite transparent individually. When two of them are placed together, however, the degree of transparency of the combination depends upon the relative orientation of the polarizers. The transmission of light can be varied from high to low, simply by rotating one polarizer with respect to the other. See also: Polarized light
Chemical effects
When light is absorbed by certain substances, chemical changes take place. This fact forms the basis for the science of photochemistry. Photosynthesis, the process by which plants produce relatively complex substances such as sugars in the presence of sunlight, is but one example of the all-important response of plant and animal life to light. See also: Photochemistry; Photosynthesis
Light pressure
When light strikes an object, it exerts a pressure on its surface that is proportional to the light intensity. An example of the action of such pressure is deflection of comet tails illuminated by solar radiation. This phenomenon has found an application in laser cooling of atoms and molecules. See also: Comet; Laser cooling; Radiation pressure
Descriptions of light
Light displays a wave-particle duality, a fundamental tenet of quantum mechanics in which every particle also has a wave nature and every wave also has a particle nature. Given the physical situation or type of observation, light can act either like a wave or like a particle. Overall, phenomena involving light may be classed into three groups: electromagnetic wave phenomena, quantum (or particle/corpuscular) phenomena, as well as relativistic effects. The relativistic effects appear to influence similarly the observation of both corpuscular and wave phenomena. See also: Quantum mechanics; Relativity; Wave-particle duality
Wave phenomena
Interference and diffraction, mentioned earlier, are the most striking manifestations of the wave character of light. Their fundamental similarity can be demonstrated in a number of experiments. The wave aspect of the entire spectrum of electromagnetic radiation is most convincingly shown by the similarity of diffraction pictures produced on a photographic plate, placed at some distance behind a diffraction grating, by radiations of different frequencies, such as x-rays and visible light. The interference phenomena of light are, moreover, very similar to interference of electronically produced microwaves and radio waves.
Polarization demonstrates the transverse character of light waves. Further proof of the electromagnetic character of light is found in the possibility of inducing, in a transparent body that is being traversed by a beam of plane-polarized light, the property of rotating the plane of polarization of the beam when the body is placed in a magnetic field. See also: Faraday effect
The fact that the velocity of light had been calculated from electric and magnetic parameters (permittivity and permeability) was at the root of Scottish physicist James Clerk Maxwell's discovery that light consists of electromagnetic waves. He wrote in 1865 that “light, including heat and other radiations if any, is a disturbance in the form of waves propagated … according to electromagnetic laws.” Finally, the observation that electrons and neutrons can give rise to diffraction patterns quite similar to those produced by visible light has made it necessary to ascribe a wave character to particles. See also: Electron diffraction; Neutron diffraction
Quantum phenomena
In its interactions with matter, light exchanges energy only in discrete amounts, called quanta. This fact is difficult to reconcile with the idea that light energy is spread out in a wave, but is easily visualized in terms of corpuscles; that is, by the particles of light called photons. See also: Photon
Blackbody (heat) radiation
The radiation from theoretically perfect heat radiators, called blackbodies, involves the exchange of energy between radiation and matter in an enclosed cavity. The observed frequency distribution of the radiation emitted by the enclosure at a given temperature of the cavity can be correctly described by theory only if it is assumed that light of frequency ν is absorbed in integral multiples of a quantum of energy equal to hν, where h is a fundamental physical constant called Planck's constant. This startling departure from classical physics, which proposed that energy consists of fundamental, discrete units called quanta, was made by German physicist Max Planck early in the twentieth century. See also: Heat radiation
Photoelectric effect
When a monochromatic beam of electromagnetic radiation illuminates the surface of a solid (or less commonly, a liquid), electrons are ejected from the surface in the phenomenon known as photoemission, or the external photoelectric effect. The kinetic energies of the electrons can be measured electronically by means of a collector which is negatively charged with respect to the emitting surface. It is found that the emission of these photoelectrons, as they are called, is almost instantaneous, and independent of the intensity of the light beam, even at very low light intensities. This fact excludes the possibility of accumulation of energy from the light beam until an amount corresponding to the kinetic energy of the ejected electron has been reached. See also: Photoelectric devices
Compton effect
The scattering of x-rays by the lighter elements is caused by the collision of x-ray photons with electrons. Under such circumstances, both a scattered x-ray photon and a scattered electron are observed, and the scattered x-ray has a lower frequency than the impinging x-ray. The kinetic energies of the impinging x-ray, the scattered x-ray, and the scattered electron, as well as their relative directions, are in agreement with calculations involving the conservation of energy and momentum. See also: Compton effect; X-rays
Quantum theories
The need for reconciling Maxwell's theory of the electromagnetic field, which describes the electromagnetic wave character of light, with the particle nature of photons, which demonstrates the equally important corpuscular character of light, has resulted in the formulation of theories that present a unified treatment of the wave and the corpuscular picture. These theories incorporate the theory of quantum electrodynamics, first set forth by English theoretical physicist Paul Dirac and others, with the earlier quantum mechanics of French physicist Louis de Broglie and others.
Dirac's synthesis of the wave and corpuscular theories of light is based on rewriting Maxwell's equations in a Hamiltonian form resembling the Hamiltonian equations of classical mechanics, formulated by Irish mathematician William Rowan Hamilton. Using the same formalism involved in the transformation of classical into wave-mechanical equations by the introduction of the quantum of action, Dirac obtained a new equation of the electromagnetic field. The solutions of this equation require quantized waves, corresponding to photons. The superposition of these solutions represents the electromagnetic field. The quantized waves are subject to Heisenberg's uncertainty principle. The quantized description of radiation cannot be taken literally in terms of either photons or waves, but rather is a description of the probability of occurrence in a given region of a given interaction or observation. See also: Hamilton's equations of motion; Nonrelativistic quantum theory; Quantum electrodynamics; Quantum field theory; Quantum mechanics; Relativistic quantum theory; Uncertainty principle
Relativistic effects
The Doppler effect for sound is familiar as the change in pitch of an ambulance's siren as it approaches and then recedes from a listener. As with sound waves, an optical Doppler effect also occurs for light on account of its wavelike nature; measured characteristics including wavelength, frequency, velocity, and the direction of the radiation in a light beam are affected by a relative motion of the light source with respect to the observer of the light. Additional effects, however, arise from the special and general theories of relativity, proposed by Einstein in 1905 and 1916, respectively. The first concerns the recessional velocity of distant galaxies as the universe expands affecting measurements of light, while the latter describes how gravitational potential also affects these quantities. See also: Doppler effect; Relativity
Cosmological and gravitational redshifts are displacements of spectral lines toward the red (lower-energy) end of the spectrum. The cosmological redshift, a staple of cosmology and astrophysics used to measure distances to objects throughout the universe, results from the expansion of space between these objects and the Earth-centric observers. The gravitational redshift, or Einstein shift, has been measured, for instance, in spectra from white dwarf stars. It results from strong gravitational fields slowing down periodic processes, shifting the spectral lines in atoms' emissions to longer wavelengths. See also: Gravitational redshift; Redshift; White dwarf star